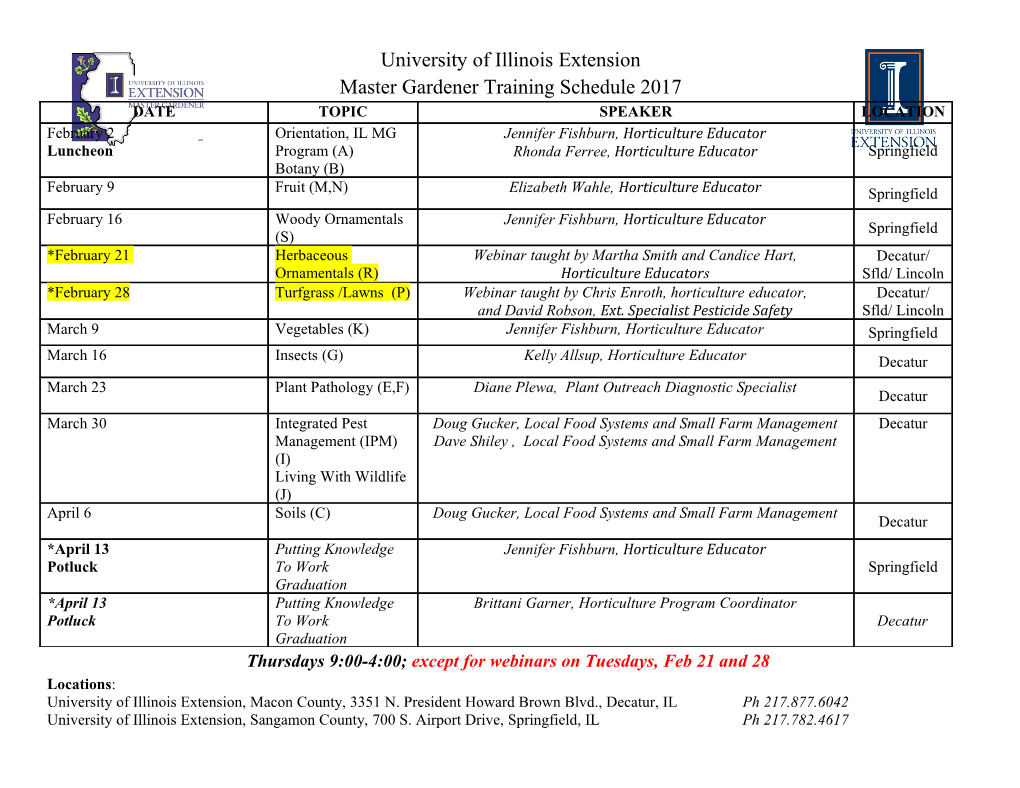
Radiation Tests of Semiconductor Detectors Valery Chmill KUNGL TEKNISKA HOGSKOLAN¨ Fysiska Institutionen Stockholm 2006 Valery Chmill: Radiation Tests of Semiconductor Detectors Abstract This thesis investigates the response of Gallium Arsenide (GaAs) de- tectors to ionizing irradiation. Detectors based on ¼-º junction formed by deep level centers doping. The detectors have been irradiated with 137Cs γ¡rays up to 110 kGy, with 6 MeV mean energy neutron up to approximately 6 ¢ 1014 n/cm2, with protons and mixed beam up to 1015 p/cm2. Results are presented for the effects on leakage currents and charge collection efficiencies for minimum ionizing electrons and alpha particles. The signal from minimum ionizing electrons was well separated from the noise even after the highest delivered exposures and the diodes are thus still operational as detectors. Saturation of the ef- fects of radiation damage is observed in both the I-V characteristics and charge collection efficiency measurements. The requirements for detectors e.g. at present and planned hadron colliders is very high in terms of radiation hardness. Detectors for tracking applications close to the interaction point will receive charged particle doses in the range of 110 kGy and fast neutron fluences of 1014 n/cm2 during the lifetime of an experiment. In this thesis it is confirmed that GaAs detectors are radiation resistant to neutron ir- radiation for fluences up to 1015 n/cm2 and that GaAs detectors are feasible as inner trackers. Most of this work was performed in the framework of the RD8 collaboration at CERN. °c Valery Chmill 2006 TRITA-FYS-2006:45 ISSN 0280-316X ISRN KTH/FYS/–06:45–SE ISBN 91-7178-401-2 Contents 1 Introduction 3 1.1 Background . 3 1.2 Aims of Work . 4 1.3 Properties of Semiconductor . 5 1.4 Band Structure . 5 1.5 Charge Carrier Mobility and Drift Velocity . 8 1.6 Classification of Defects in Semiconductor . 8 1.7 Author’s Contribution . 10 1.7.1 List of publications included in thesis. 11 1.7.2 List of publications not included in thesis. 12 2 Description of Investigated Structures 14 2.1 Technology . 15 2.1.1 Diffusion . 15 2.1.2 Epitaxy . 16 3 Response of GaAs detectors to Ionizing Irradiation 19 3.1 Measuring bench . 21 3.2 Response of the GaAs samples to radioactive sources . 22 3.3 Signal formation mechanism and estimations of charge collection time . 25 3.4 Temperature dependence of the properties of ¼-º struc- tures . 31 3.5 γ–ray irradiation of GaAs structures . 34 4 Neutron Irradiation of GaAs Structures 37 4.1 Description of the experimental method . 37 4.2 Irradiation induced defects and test structures properties 39 1 2 Contents 4.3 Sensitivity of GaAs structures to minimum ionizing beta particles . 44 4.4 Conclusion . 47 5 Irradiation in Mixed Beam at CERN 48 5.1 Introduction . 48 5.2 Investigated structures . 49 5.3 Experimental details . 50 5.4 Exposure in mixed beam, basic results . 51 5.5 Signal estimation . 56 6 Microstrip Detectors Test 57 6.1 Main parameters of the microstrip detectors . 58 6.2 Experimental set-up . 60 6.3 The beam test results . 61 6.4 Investigation of radiation hardness . 65 7 GaAs detectors for ®-particles and heavy ions spectrom- etry 68 7.1 Introduction . 68 7.2 Detector manufacture technology . 69 7.3 Electrical characteristics . 69 7.4 Detector performance for ®-particles . 72 8 X-ray Irradiation of Silicon Detectors 76 8.1 Experimental layout . 77 8.2 Results and discussions . 79 8.3 Summary . 86 9 Summary and Outlook 87 9.1 Comparison with prediction from Local Charge Neutral- ity model . 87 9.2 Rate of Irradiation . 89 9.3 Conclusion . 90 10 Acknowledgments 92 Chapter 1 Introduction 1.1 Background The operation of semiconductor detectors in harsh radiation environ- ments depends strongly on the radiation hardness of the device. One such environment is the CERN Large Hadron Collider (LHC). Here the inner tracker detectors close to the interaction point will receive charged particle doses in the range of 110 kGy and fast neutron fluences of 1014 n/cm2 during the lifetime of an experiment. Applications with harsh radiation environments exist in a wide range of fields from ex- periments with high charged particle multiplicity to proton and nuclei irradiation therapy. The basic damage mechanisms in semiconductors are conveniently separated into two categories: a) Bulk effects, which are manifestations of the displacement of atoms from their normal sites in the crystal lattice. b) Surface effects, which are long-term ionization effects in the in- sulators connected with the active semiconductor region. Modern electronics is based on semiconductor structures formed by the introduction of impurities into semiconductors, thus creating cen- ters with shallow energy levels (see later in this chapter). The Siberian Institute of Technology in Tomsk, Russia, has over the last twenty years adopted a non-traditional approach to create device structures through doping semiconductors with impurities which create centers with deep 3 4 Chapter 1. Introduction energy levels. The resulting, so called ¼-º structures enabled new de- vices, since these structures allows to form high ohmical intrinsic-like GaAs material. In this thesis, the effects of bulk damage due to gamma, neutron, proton and pion irradiation on the properties of GaAs detectors in terms of current as a function of voltage (I-V), capacitance as a func- tion of reverse bias voltage (C-V) measurements and charge collection efficiency degradation are experimentally investigated. The thesis also includes a study of recovery effects of a Si-detectors by voltage training from X-ray irradiation. 1.2 Aims of Work The aims of this work are to enhance the understanding of GaAs with deep level dopants from an experimental physics point of view and to study the radiation hardness of GaAs for high energy physics applica- tions. More specifically this involved: ² investigation of differences between industry-standard, SI-U (Semi- Insulating, Undoped), LEC (Liquid-Encapsulated Czochralski [1]) and epitaxially-grown GaAs layers ² provide input to optimize fabrication technology for simple pad and microstrip detectors production ² evaluation of the radiation hardness of GaAs detectors to charged particles, neutrons and gamma ² testing of commercial and laboratory pad and microstrip detec- tors to LHC specification with LHC speed read-out electronics ² development of methods for enhancing the detection efficiency for ionizing radiation. ² investigate the feasibility of GaAs detectors as ®-particles spec- troscopy detectors 1.3. Properties of Semiconductor 5 1.3 Properties of Semiconductor Figure 1.1: Unit cube of GaAs crystal lattice [2]. The first synthesization of GaAs was reported in 1929 by Gold- schmidt, and the first reported electronic properties of III-V (3 and 5 valence electrons respectively) compounds as semiconductors appear in 1952 [3]. Two sublattices build up the crystal, each of them consists of a so called face centered cubic lattice (fcc). The off-set between the two lattices is equivalent to half of the diagonal of the fcc cube. This structure is normally referred to as zinc blende. All semiconduc- tor compounds from group III-V and II-VI have the same crystalline structure; Si and Ge (group IV) have the same crystalline structure as diamond. Both the diamond lattice and the zinc-blende lattice are cubic lattices. Figure1.1 shows a unit cube for GaAs. In Table 1.1 a list of fundamental properties and characteristics is given for GaAs and Si [4]. 1.4 Band Structure Electrons in isolated atoms can have only certain discrete energy values. When isolated atoms are put together in a crystal, the electrons are not restricted to single energy levels, but ranges of energies are allowed. 6 Chapter 1. Introduction Property Units GaAs Si Crystal structure Zinc blende Diamond Lattice constant [A]˚ 5.6533 5.43095 Density [g/cm3] 5.32 2.328 Atomic density [atoms/cm3] 4.5¢1022 5¢1022 Molecular weight 144.64 28.09 El.-hole pair creation energy [eV/pair] 4.3 3.7 Dielectric constant 12.85 11.9 Band gap at 300K [eV] 1.42 1.14 Intrinsic carrier conc. [cm¡3] 1.7¢106 1.45¢1010 Electron mobility (undoped) [cm2/V¢s] 8500 1500 Hole mobility (undoped) [cm2/V¢s] 400 450 Melting point [oC] 1238 1415 Table 1.1: Room-temperature properties of GaAs and Si. Those bands are referred to as valence and conduction bands (Fig- ure 1.2). Figure 1.2: Energy band diagram for GaAs [5]. These bands are separated by an energy band gap, which is the main parameter for the semiconductor material. At low temperatures, there is little thermal energy available to push valence electrons across this gap, and the semiconducting material acts as an insulator. At higher temperatures though, the ambient thermal energy becomes sufficient 1.4. Band Structure 7 to force electrons across the gap, and the material will conduct elec- tricity. The probability of an electron having enough energy to make the transition is given by the Fermi distribution function 1.1. 1 f(E) = (1.1) eE¡EF =kT + 1 The Fermi level shown on Figure 1.2 is the energy level at which the probability is equal to one half. For intrinsic semiconductors, the Fermi level is in the center of the band gap. For undoped GaAs, the energy band gap at room temperature is 1.42 eV and for Si 1.14 eV. The energy band diagram is referenced to the vacuum potential [6,7]. Figure 1.3: Energy band structure of Si and GaAs [5]. GaAs is a direct band gap semiconductor, Figure 1.3. This means that transitions between bands require only a change in energy, and no change in momentum.
Details
-
File Typepdf
-
Upload Time-
-
Content LanguagesEnglish
-
Upload UserAnonymous/Not logged-in
-
File Pages106 Page
-
File Size-