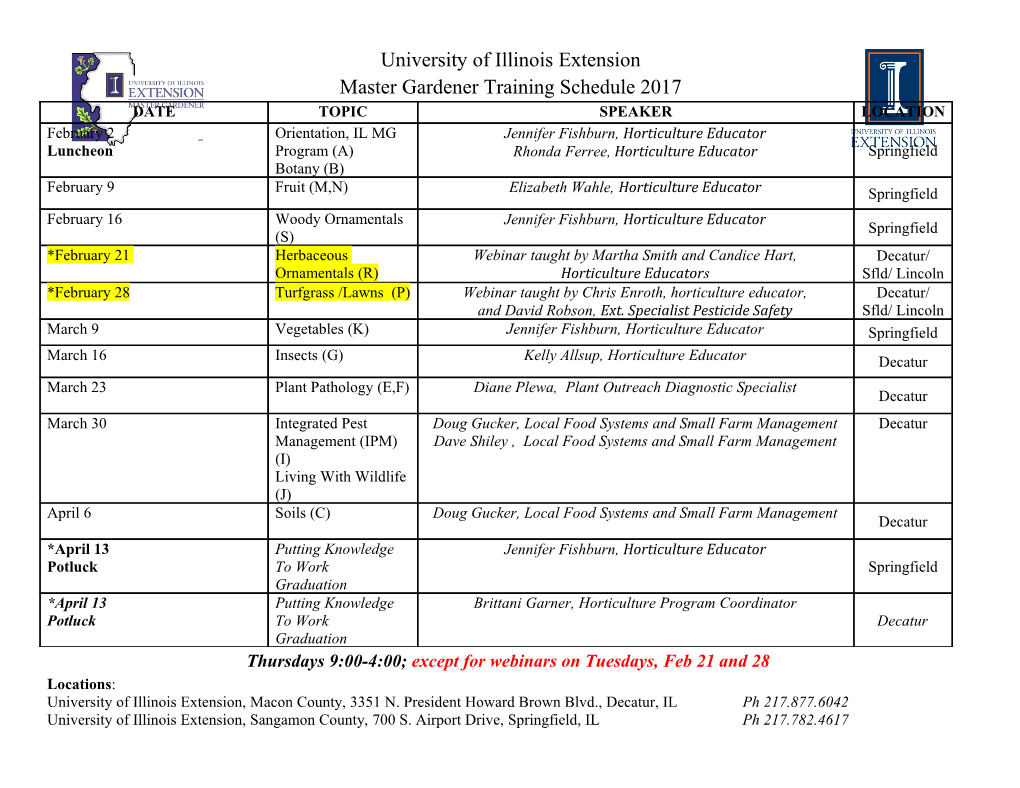
INTRODUCTION: The twentieth century saw the biggest increase in the population worldwide [1]. Recently, India has crossed the figure of 121 billion in terms of population. This will naturally increase the consumption of energy as it is the paramount importance for an industrialized economy. Currently, to fulfill the needs of energy, people depend mainly on the fossil fuels i.e. on coal and natural gas. A robust growth in the consumption of the fossil fuel has been seen and this was reported in the annual report i.e. Statistical Review of World Energy, published on June 2011. The consumption of coal, oil and natural gas is found to be increased by 7.6%, 3.1% and 7.4% respectively in the year 2010 as compared to the previous year [2]. It is also predicted that the global average temperature will rise from 1.4 to 5.8 °C by year 2100 and continue to rise long after that [3]. This rise is mainly due to the rapid increase in the emission of greenhouse gases (CO 2 and others) in the atmosphere, which are emitted by the burning of the fossil fuels [4]. To fulfill the energy demand is not the only problem linked with the increase in the population. Beside this, waste generation is also a problem. In India, daily tonnes of waste are generated. Daily 6000 tonnes of waste is generated alone in Delhi and only 62% of the total waste is recycled as against 5,800 tonnes in Mumbai, 2,800 in Bangalore, 2,675 tonnes in Chennai and 4,000 tonnes in Kolkata [5]. Wastes may be of two types i.e. solid waste and liquid waste and both are creating problems as the disposal of the waste is causing nuisance to the society. Water which has been adversely affected by the influence of anthropogenic sources is considered as wastewater [6]. Waste management [7] is becoming one of the key problems of the modern world, an international issue that is intensified by the volume and complexity of domestic and industrial waste discarded by society. Agra, the city of Taj is also not devoid of this waste problem. Taj city is not only famous for Taj Mahal but also for the petha sweet prepared in the narrow streets of Noori Darwaja. This sweet is prepared from the fruit 'Ash Gourd', the botanical name of which is Benincasa hispida and is commonly known in India as Petha. This has also been named as Kushmand in ancient ayurveda, and is believed to have remarkable curative properties [8]. There are around 500 petha industries units running in Agra [8-10]. These petha industries generate about 30-35 tonnes of solid waste daily [11-12] and uses coal for processing the sweet. 1 Supreme Court has banned the use of coal in Agra but most of these units are using it. To avoid the effect of burning of coal (used by petha industry) Development Authority wants to relocate the petha industries at the outskirts of the city [9 & 13]. In addition to this, petha industries use lime water to wash the petha fruit and then drain it as such untreated. This wastewater discarded is highly alkaline. Clean Technology Initiative (CTI) project started a “Waste-to-energy” [12 & 14] program to generate methane gas from the petha waste which can replace the coal for petha making. But it seems that now days this process is not in action as tons of waste is found nearby these units and create problem to the local people. Biofuel production [15-20] from the biodegradable waste [21] plays the dual role as this can be used for both i.e. waste disposal and for energy production. Biological processes are considered as the clean and sustainable method to produce hydrogen. There are mainly three methods by which hydrogen can be produced biologically i.e. biophotolysis of water, photofermentation and dark fermentation [22]. In direct biophotolysis, cyanobacteria decompose water to generate hydrogen and oxygen in the presence of light. In photofermentation [23-24], anoxygenic photoheterotrophic bacteria utilize organic feedstock to produce hydrogen in the presence of light. In dark fermentation (DF) also known as anaerobic degradation [25-26], anaerobic heterotrophic bacteria utilize organic feedstock without any light to produce hydrogen [22, 27- 29]. To stabilize the waste and convert it into energy anaerobic degradation [30-33] is the process which is mainly used and it acts as the most promising method for hydrogen production [34]. This process is mainly classified into four steps i.e. Hydrolysis [35] : A chemical reaction where particulates are solubilized and large polymers converted into simpler monomers; Acidogenesis [36] : A biological reaction where simple monomers are converted into volatile fatty acids; Acetogenesis [37] : A biological reaction where volatile fatty acids are converted into acetic acid, carbon dioxide, and hydrogen; and Methanogenesis [38 & 39] : A biological reaction where acetate and hydrogen are converted into methane and carbon dioxide, while hydrogen is consumed. Anaerobic digestion is mainly based on two important steps i.e. 2 Biohydrogen generation [40] : C6H12 O6 + 2H 2O 4H 2 + 2CH 3COOH + 2CO 2 Methane formation [41] : CO 2 + 4H 2 CH 4 + 2H 2O CH 3COOH CH 4 + CO 2 Both hydrogen and methane are important fuel but hydrogen has more advantage over methane as it is clean, safe, renewable and high calorific value [42]. So to increase the production of the hydrogen some pretreatment methods are used to block the reaction at the methanogenesis step and hence the production of hydrogen is increased. Acetate can also be converted into hydrogen and carbon dioxide. There is another method to produce hydrogen, which is coupled with the electricity production using microbial fuel cell (MFC) in which chemical energy changes into the electrical energy under anaerobic conditions [43-45]. Electrons produced by the bacterial oxidation of the organic matter present in the waste are transferred to the anode (negative terminal) and flow to the cathode (positive terminal) linked by a conductive material containing a resistor, or operated under a load (i.e., producing electricity that runs a device) [46]. Chemical mediators, such as neutral red or anthraquinone-2,6-disulfonate (AQDS), can be added to the system to allow electricity production by bacteria unable to otherwise use the electrode [47]. If no exogenous mediators are added to the system, the MFC is classified as a “mediator- less” MFC even though the mechanism of electron transfer may not be known [48]. On the basis of the design, MFCs are classified into two types i.e. single chambered microbial fuel cell (SCMFC) in which cathode and anode are present in the same chamber and dual chambered microbial fuel cell, anode and cathode are separated in two chambers which are separated by a permeable membrane used for proton exchange like Nafion, ultrex etc. [47 & 49]. Related to MFC, microbial electrolysis cell (MEC) partially reverses the process to generate hydrogen or methane from organic material by applying an electric current. 3 LITERTAURE CITED: Biofuel production from the waste (solid and liquid) has been studied by different investigators and they have reported the various results. The effect of the various inoculum pretreatment methods on the production of the biofuel was also reported. In addition to this the working of the microbial fuel cell was also studied by several researchers and the effect of the variations in the components of the cell has been studied recently. Some of these studies are discussed below. Zhu et al. [50] studied the characterization and the biohydrogen production using food waste (FW), primary sludge (PS) and the waste activated sludge (WAS). The different combinations of the waste was studied (FW+PS, FW+WAS and FW+PS+WAS) and in different ratio. The mixing ratio of 1:1 was found to be the best as compared to the other ratios studied. A hydrogen yield of 112 mL/g volatile solid (VS) added was obtained from a combination of FW, PS and WAS. This yield was equivalent to 250 mL/g VS added if only FW contributed to hydrogen production. Hydrogen production from the corn syrup waste and the effect of the organic loading rate on the hydrogen production has been studied [51]. Methanogenic activity was also inhibited by heating 0 the mixed culture at 70 C for 30 minutes. Hydrogen production rate increased from 10 L H 2/L·d to 34 L H 2/L·d with the increase of organic loading rate (OLR) from 26 to 81 gCOD/L·d, while a maximum hydrogen yield of 430 mL H 2/gCOD was achieved in the system with an overall average of 385 mL H 2/gCOD . In another study the thermophillic anaerobic hydrogen production using kitchen waste was estimated [52]. The production of the hydrogen in the different seasons was studied as the chemical oxygen demand varies in the different seasonal dietary habits. The hydrogen -1 -1 production rate and yield at volumetric loading rate (VLR) of 28 g-COD L day were 1.0 L-H2 -1 -1 -1 L day and 1.7mmol-H2 g-COD respectively, which were higher than at VLR of 19 g-COD/L- day. Gomez et al. studied the hydrogen production through different methods [53]. Dark fermentation has two main advantages: fulfilling requirements for mild operational conditions and gaining benefit from the residual biomass. It generated a hydrogen yield of 33%. Photofermentation and 4 bioelectrochemical systems (BES) are the processes capable of converting fermentation sub- products (like acetic acid) into hydrogen. Hydrogen production in the combined light and dark conditions from the ground wheat powder solution (10g L -1) has also been reported by some researchers [54].
Details
-
File Typepdf
-
Upload Time-
-
Content LanguagesEnglish
-
Upload UserAnonymous/Not logged-in
-
File Pages23 Page
-
File Size-