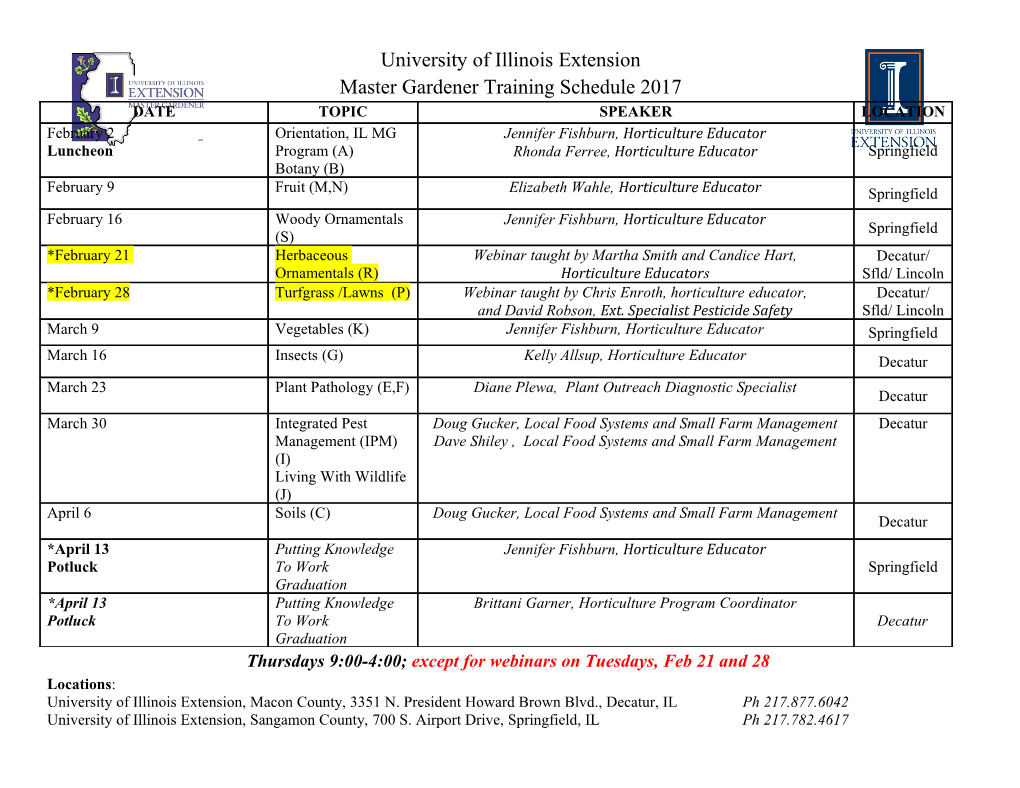
Magneto-strictive, Magneto-rheological & Ferrofluidic Materials Corso Materiali intelligenti e Biomimetici 2/04/2020 [email protected] Magnetic Properties Magnetic Permeability: indicates how easily a material can be magnetized. It is a constant of proportionality that exists between magnetic induction and magnetic field intensity. µr =µ/µ0 (relative permeability) -6 µ0=1.26 x 10 H/m in free space (vacuum) • Materials that cause the lines of flux to move farther apart, resulting in a decrease in magnetic flux density compared with a vacuum, are called diamagnetic; • materials that concentrate magnetic flux are called paramagnetic (non-ferrous metals such as copper, aluminum); • materials that concentrate the flux by a factor of more than ten are called ferromagnetic (iron, steel, nickel). Magnetic Properties • Diamagnetic: dipoles formed under the applied field H-> oriented in an opposite direction with respect to the applied H (no net magnetization) • Paramagnetic: existing random dipoles -> oriented in the same direction of the applied H (small magnetization) • Ferromagnetic: existing alligned dipoles -> oriented in the same direction of the applied H (large magnetization) domains Magneto-strictive materials (MS) Ferromagnetic materials have a structure that is divided into domains (regions of uniform magnetic polarization). When a magnetic field is applied, the domains rotate causing a change in the material dimensions. Magnetostriction is a property of ferromagnetic materials that causes them to change in shape of materials under the influence of an external magnetic field. Magnetostriction is a reversible exchange of energy between the mechanical and the magnetic domain (magneto-mechanical coupling). Magnetostriction The reason that a rotation of the magnetic domains of a material results in a change in the materials dimensions is a consequence of magneto-crystalline anisotropy: it takes more energy to magnetize a crystalline material in one direction than another. If a magnetic field is applied to the material at an angle to an easy axis of magnetization, the material will tend to rearrange its structure so that an easy axis is aligned with the field to minimize the free energy of the system. Since different crystal directions are associated with different lengths this effect induces a strain in the material. Curie Temperature When the material is above its Curie temperature, it exists in a paramagnetic state and is composed of unordered magnetic moments in random orientations. On cooling below the Curie temperature (spontaneous polarization), the material becomes ferromagnetic, and the magnetic moments become ordered over small volumes (domains). However, because the domains are randomly oriented, the net magnetization of the material is zero. Field-induced Magnetostriction Applying a magnetic field below the Curie temperature, leads to domains re-orientation in the direction of magnetic field and to a global deformation of the material. Strain vs. Magnetic field Region 0-1: domains start to align (non linear relationship) Region 1–2: almost linear relationship between strain and magnetic field (most devices are designed to operate in this region). Region 1–2: the relationship becomes non-linear since most of the magnetic domains have become aligned with the magnetic field direction (the increase of magnetic field is no more proportional with the strain). Beyond point 3: saturation (all the magnetic domains have become aligned with the magnetic field and no further strain is achievable) Strain vs. Magnetic field (2) When a magnetic field is established in the opposite direction, the negative field produces the same elongation in the magnetostrictive material (strain direction is independent from magnetic field polarity ).For its shape, the curve strain vs. magnetic field curve is referred as butterfly curves. The positive (expansion) or negative magnetostriction (contraction) of the material depends on material intrinsic properties. Magnetostriction in pre-stressed conditions If the material is pre-stressed, the recoverable strain is larger. (Main) Magnetostrictive Effects The Joule Effect is related to material deformation under an external magnetic field. The Villari Effect is a change of the magnetic properties of a material when subjected to a mechanical stress. The change in the magnetic flux density can be detected by a pickup coil and is proportional to the level of the applied stress. Sensor Rod 3D example Terfenol rod ‘33-mode’ Numerical Example: actuator terfenol rod Example of MS materials • Cobalt exhibits a room-temperature magnetostriction of 60 microstrains in a field of 160 kA/m . • Cobalt alloys: Terfenol-D (Ter for terbium, Fe for iron, NOL for Naval Ordnance Laboratory, and D for dysprosium) exhibits about 2000 microstrains in a field of 160 kA/m at room temperature and is the most commonly used engineering magnetostrictive material. MS properties Application example: Linear MS actuator Application example(2): MS inchworm motor When one of the coils is energized, the section of rod directly exposed to the magnetic field elongates. As the field is removed, the rod clamps itself again inside the stator but at a distance d to the left of the original position. As the remaining coils are energized sequentially, the rod moves in the direction opposite to the sweeping field. The direction of motion is changed by inverting the coil activation sequence. Magneto-rheological (MR) materials Micrometric (0.1–10 µm range) ferromagnetic particles randomly dispersed in oil or water. MR fluid particles are too dense for Brownian motion to keep them suspended, thus surfactants are included in the mixture to avoid the settling of the suspended particles. MR materials: behaviour When subjected to a magnetic field, the ferromagnetic particles align their magnetic dipoles along the lines of magnetic flux. These microscopic chains have a the macroscopic effect to change fluid viscosity to the point of becoming a viscoelastic solid. The main advantage of MR materials is that the amount of dissipated energy of the system is simply controllable by acting on the coil current and the system can provide semi-active damper behaviour. MR materials: rheological properties The MR fluid behaves following a Bingham law with a yield shear stress (critical stress at which the material start to flow). In the MR fluids, the value of the yield stress is controlled by the applied magnetic field: the larger the field, the higher the yield stress and thus the force the material can withstand without flowing. Lumped parameter model 1 Lumped parameter model 2 Working modes a) Flow-mode exploits the flow between two fixed walls, the magnetic field is normal to the flow direction and is typical for linear damper applications. b) Shear-mode is mainly used in rotary application such as brakes and clutches and the fluid is constrained between two walls which are in relative motion with the magnetic field normal to the wall direction. c) Squeeze-mode is used mainly for bearing applications, is able to provide high forces and low displacements having the magnetic in the same direction of the applied force. H H H Calcolo coefficiente smorzamento Shear-mode operation Flow-mode operation c=c0(1+Bi) c=c0 c0_SM=μα c0_FM=12μα Commercial MR & Limitations • High density, due to presence of iron, makes them heavy. However, operating volumes are small, so while this is a problem, it is not insurmountable. • High-quality fluids are expensive. • Fluids are subject to shear-thickening (non linear behaviour) after prolonged use and need replacing. • Settling of ferro-particles can be a problem for some applications. Example of application: Controllable Linear Dampers The desired level of damping can be obtained varying the magnetic field in an orifice between two separated MR fluid chambers. The orifice acts like a magnetic valve for the fluid, regulated by the current and thus exploits the MR fluid in flow mode. Ferrofluids (FF) Differently from MR fluids, FF particles are primarily nanoparticles that are suspended by Brownian motion and generally will not settle under normal conditions. Ferrofluid is superparamagnetic, which means that they behave like magnets only in the presence of a magnetic field. This property is found only at the nanoscale. At the macroscale, ferromagnetic materials are permanently magnetic. FF applications FF keeps its fluidity even if subjected to strong magnetic fields. Most applications of magnetic fluid are based on the following of its properties: • It goes to where the magnetic field is strongest and stays there; • It absorbs electromagnetic energy at convenient frequencies and heats up. Biomedical Applications: 1) Magnetic drug targeting; 2) Hyperthermia; 3) Contrast enhancement for Magnetic Resonance Imaging; 4) Magnetic separation of cells. FF - Biomedical Applications 1. Magnetic drug targeting a ferrofluid bounded drug is injected in a cancer tumor and there it is kept during some time (≈ one hour) by a suitably focused magnetic field. The amount of drug necessary is much less than what would be necessary if it were dispersed in the whole body (dose reduction). When the magnetic field is turned off the not metabolised drug will disperse in the body, but, since the total amount is very small, there will be practically no side effects. 2. Hyperthermia The property of ferrofluids of absorbing electromagnetic energy at a frequency that is different from the frequency at which water absorbs energy allows one to heat up a localized portion of a living body, where ferrofluid has been injected, for example a tumor, without heating at the same time the surrounding parts of the body. FF - Biomedical Applications 3. Contrast enhancement for Magnetic Resonance Imaging (MRI) If magnetic particles from a biocompatible FF are selectively absorbed by some kind of tissue, this will become very clearly visible by MRI. Dextran coated iron oxide NP are biocompatible and are excreted via the liver after the treatment. They are selectively taken up by normal cells with respect to tumor cells, which do not have an effective reticuloendothelial system. This makes them distinguishable from the surrounding healthy cells.
Details
-
File Typepdf
-
Upload Time-
-
Content LanguagesEnglish
-
Upload UserAnonymous/Not logged-in
-
File Pages37 Page
-
File Size-