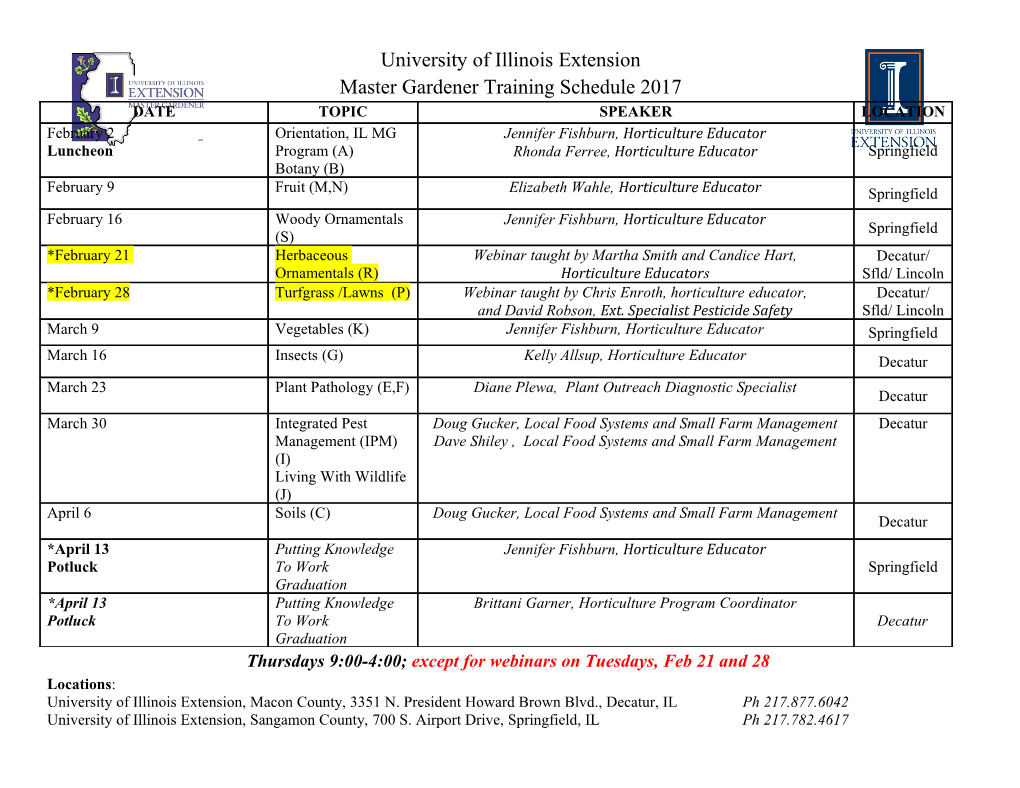
Direct Look at the Electric Field in Ketosteroid Isomerase and its Variants Matthew R. Hennefarth† and Anastassia N. Alexandrova*,†,‡ †Department of Chemistry and Biochemistry, University of California, Los Angeles, 607 Charles E. Young Drive East, Los Angeles, CA 90095-1569, USA ‡California NanoSystems Institute, University of California, Los Angeles, 570 Westwood Plaza, Los Angeles, California 90095-1569, USA E-mail: *[email protected] Phone: +1 310 825-3769 Abstract Enzymes catalyze a number of reactions with high efficiency and stereoselectivity. It is thought that strong, direct, and permanent electric fields within the active site of the enzyme contribute to the superb catalytic efficiency of enzymes. This effect is called electrostatic preorganization. Most often, electrostatic preorganization is analyzed by evaluating the local electric field at discrete points, such as a bond center, using, for example, vibrational Stark spectroscopy. However, the protein macromolecule creates a significantly more complicated heterogeneous electric field that affects the entire active site, whose total change density thus gets perturbed, with the implications for the catalytic mechanism. We present a global distribution of streamlines method to analyze the topology of the heterogeneous electric fields in within an enzyme active site. We focus on ketosteroid isomerase (KSI), an enzyme known to produce a field on the order of 100 MV/cm along the critical carbonyl bond in the steroid substrate. We investigate how mutations known to cause activity changes, as well as applied small external electric fields perturb the electric fields in the KSI active site. Where classical single-point analysis failed, using our method allowed us to properly correlate global changes in the electric field to changes in the reaction barrier. We were able to show that topologically similar local electric fields had similar reaction barriers. Keywords KSI, Electrostatic Preorganization, Electric Field, Vector Field, Enzymes 1 Enzymes are an essential part of bioloGy, chemistry, and medicine. They can catalyze chemical reaction with incredible efficiency and stereoselectivity under physioloGical conditions, and thus are desirable tools for catalyzing non-native reactions in a wide variety of applications. While a protein can be comprised of several hundred amino acid residues, only a small subset of these residues actually interacts with the substrate durinG the reaction. This begs the question of the necessity of the rest of the protein scaffold for catalysis. It is hypothesized that the rest of the protein contributes to catalysis by producing a highly specific and preorganized electric field that facilitates the reaction. The formation of this specific electric field falls within the idea of electrostatic preorGanization, the notion that enzymes create an electrostatically orGanized environment such that there is a reduced entropic cost to reach the rate-determininG transition state (TS).1,2 If the reaction that a protein catalyzes would instead occur, for example, in a polar solvent, there would be an entropic penalty to realign the solvent’s dipoles to stabilize the TS.1,3 The free enerGy difference between the TS and reactant determines the reaction rate; therefore, lowerinG the free enerGy barrier by removinG the entropic penalty to reach TS while simultaneously destabilizinG the reactant state would Greatly increase the reaction rate. It is thouGht that proper aliGnment of charGed amino acid residues surroundinG the active site, even at a distance, creates a permanent electric field that is optimized within the active site for the reaction. It has been shown that residues in the first and second coordination sphere contribute the most to this electric field.4–7 Hence, the protein scaffold needs to be folded in a specific way such that these residues are in the correct spatial position and orientation to optimize the effect of the electric field on the active site. It is of interest to be able to quantify a protein’s electrostatic preorganization so to better understand the functionality of natural enzymes and to improve computationally desiGned enzymes, whose startinG activity remains suboptimal.8–12 One of the most studied enzymes in the context of understandinG electrostatic preorGanization is ketosteroid isomerase (KSI) (Figure 1). Subjected to both theoretical and experimental interroGations, KSI has one of the hiGhest known unimolecular rate constants13,14 with many theoretical15– 21 and experimental22–26 studies investigating its electrostatic preorganization. KSI catalyzes the isomerization of a steroid by alterinG the position of a C=C double bond throuGh formation of a charGed enolate after a proton abstraction and reinsertinG the 2 proton two carbons away (Scheme 1). When the charGed intermediate forms, there is expected to be an increased dipole along the carbonyl bond due to a resonance-assisted decrease of the double bond character. Y32 Y57 D40 Y16 r+ D103 b+ W120 g+ Figure 1: Full KSI protein (PDB code 1OH027). Residues included in the small-scale calculations are highlighted in blue. The substrate is colored green. At right, the site is shown schematically, with red, blue, and green arrows representing the directions of the external electric fields applied to the system in this study. E-S E-I E-P deprotonation protonation Scheme 1: Reaction catalyzed by KSI including important residues along the reaction pathway. Warshel used the empirical valence bond (EVB) method to detect differinG amounts of electrostatic preorGanization dependinG on the confirmation of the substrate present in KSI;15,18 he also used the same approach to explain the catalytic efficiency of methyltransferases.28 Boxer later showed using vibrational Stark spectroscopy that KSI exerts an optimized electric field, around −144 ± 6 MV/cm, alonG the carbonyl bond to increase the stability of the first TS and the intermediate.24 Calculations of the vibrational shifts of a nitrile probe in KSI confirmed the findinGs by Boxer.17 Head-Gordon has used 3 molecular dynamic simulations to investiGate the fluctuation of the electric field at the carbonyl bond in KSI throughout the catalytic cycle.7 More recently, Sokalski has investiGated the dynamic nature of the electric field and its role in proton dislocations in the hydrogen-bond network around the substrate carbonyl. In theoretical studies, the electric field is probed at only discrete points: either the center of the bond or at the two atoms that make up the bond in question. However, the electric field in the active site is heterogeneous and more complicated than what a sinGle point can describe. At the same time, the electron density on the carbonyl is interdependent with the electron density in the rest of the active site. The entire electron density is affected by the heterogeneous electric field, which thus affects the catalyzed reaction. Previous studies have shown that external electric fields can significantly influence the reaction rates,29–33 and molecular properties, includinG chemical bonds34. No theoretical or experimental method to date actually probes the full electric field in the reGion that makes up the carbonyl bond, or the full active site. The electric field is dependent on both the electron density, �(�), and nuclear coordinates. Under the assumption that the nuclear coordinates are relatively constant across systems we miGht be interested to compare, chanGes in �(�) should reflect changes in �(�). Additionally, �(�) is a scalar function, makinG it easier to analyze the global properties of �(�). As such, the analysis of the topoloGy of �(�) throuGh quantum theory of atoms in molecules (QTAIM) has also been utilized to explore electrostatic preorGanization in KSI,16 and in the histone deacetylase 8.5 In these previous studies, we have shown that active site critical points of �(�) as well as the curvature and charge density at these critical points are good descriptors of electrostatic preorganization.5,16 Specifically for KSI, the QTAIM formalism was employed to detect chanGes in the topoloGical properties of �(�) as different uniform, external electric fields were applied to the active site of KSI. We showed how the chanGes in the QTAIM siGnature of the active site correlated stronGly with both the electric field, and the changes in the reaction barrier of KSI. Therefore, �(�) serves as a convenient scalar mediator between the reaction rate and the electric field, enablinG an indirect analysis of the electrostatic preorganization. While analysis of �(�) provides an indirect measurement of electrostatic preorGanization, it still does not map or analyze the actual non-uniform vector electric field produced by the protein. 4 Traditional theoretical treatment of the electric vector field consists of evaluating the field at singular points, disregarding the general topology of the electric field.4,7,21,35,36 Here, we introduce a method to quantify perturbations in the locally produced electric fields that incorporates the Geometry of the electric field so as to directly quantify the electrostatic preorGanization. We use KSI as a model system due to the larGe number of experimental and theoretical studies investiGatinG its electrostatic preorGanization. We showcase our method on two types of systems. In the first type, we apply external electric fields to the active site of KSI so as to decouple it from the heteroGeneous effects of the protein residues; the field affects the reaction barrier. The second
Details
-
File Typepdf
-
Upload Time-
-
Content LanguagesEnglish
-
Upload UserAnonymous/Not logged-in
-
File Pages27 Page
-
File Size-