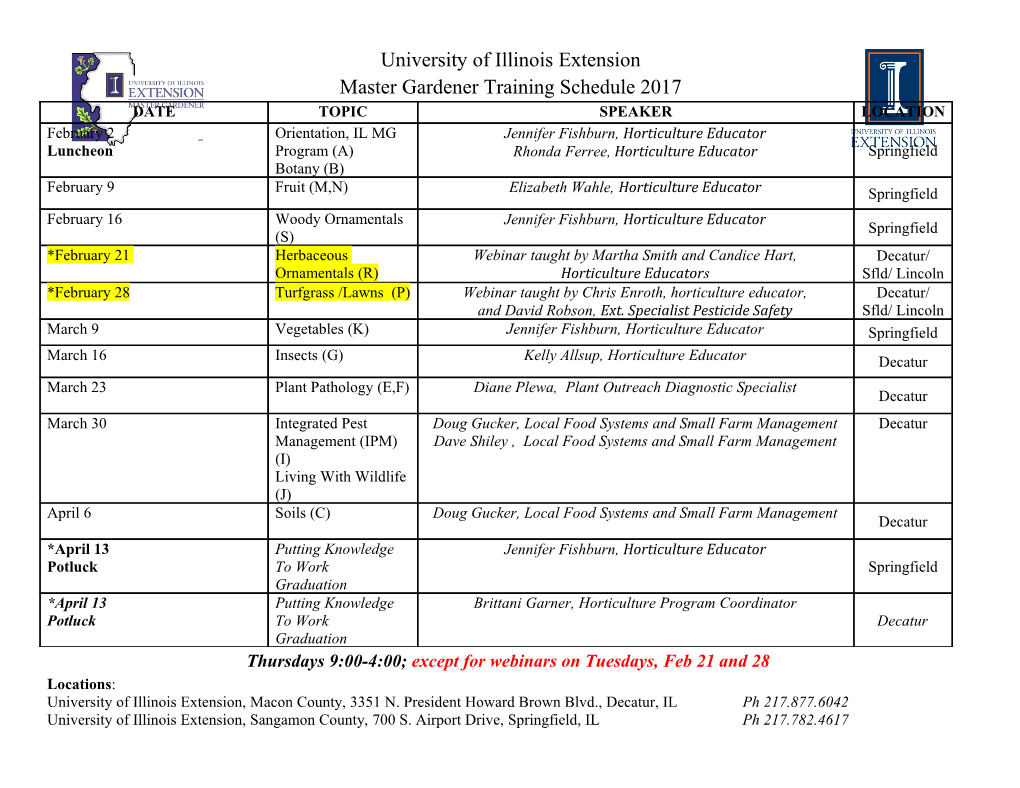
Ultrahydrous stishovite from high-pressure hydrothermal treatment of SiO2 Kristina Spektora,b, Johanna Nylenb, Emil Stoyanovc, Alexandra Navrotskyc,1, Richard L. Hervigd, Kurt Leinenweberb, Gregory P. Hollande, and Ulrich Häussermanna,1 aDepartment of Materials and Environmental Chemistry, Stockholm University, S-10691 Stockholm, Sweden; bDepartment of Chemistry and Biochemistry, Arizona State University, Tempe, AZ 85287-1604; cPeter A. Rock Thermochemistry Laboratory and Nanomaterials in the Environment, Agriculture, and Technology Organized Research Unit, University of California, Davis, CA 95616; dSchool of Earth and Space Exploration, Arizona State University, Tempe, AZ 85287-1404; and eDepartment of Chemistry and Biochemistry, Magnetic Resonance Research Center, Arizona State University, Tempe, AZ 85287-1604 Contributed by Alexandra Navrotsky, October 19, 2011 (sent for review September 2, 2011) Stishovite (SiO2 with the rutile structure and octahedrally coordi- A nated silicon) is an important high-pressure mineral. It has pre- viously been considered to be essentially anhydrous. In this study, hydrothermal treatment of silica glass and coesite at 350–550 °C near 10 GPa produces stishovite with significant amounts of H2O in its structure. A combination of methodologies (X-ray diffraction, thermal analysis, oxide melt solution calorimetry, secondary ion mass spectrometry, infrared and nuclear magnetic resonance spec- troscopy) indicate the presence of 1.3 0.2wt%H2O and NMR B C suggests that the primary mechanism for the H2O uptake is a direct hydrogarnet-like substitution of 4H for Si4, with the protons clustered as hydroxyls around a silicon vacancy. This substitution is accompanied by a substantial volume decrease for the system (SiO2 H2O), although the stishovite expands slightly, and it is only slightly unfavorable in energy. Stishovite could thus be a host for H2O at convergent plate boundaries, and in other relatively cool high-pressure environments. 1mµ 5mµ D E low temperature ∣ high-pressure synthesis ∣ hydrothermal environments ∣ multianvil technique ilica is an archetypical system for Earth and materials science. SIts complex polymorphism remains the subject of continuing study (1–3). At ambient to moderate pressures (up to 9 GPa), all forms of silica are built up of SiO4 tetrahedra, with coesite the highest pressure polymorph of this type. At higher pressures, 1mµ 2mµ dense forms containing SiO6 octahedra occur. Stishovite with the tetragonal rutile structure is stable between 9 and 50 GPa (4). Fig. 1. Photomicrograph of a partially glass-coesite transformed particle obtained at 300 °C in plane-polarized light (A, Left) and in cross-polarized Coesite and stishovite are believed to occur in silica rich parts of ’ light (A, Right). SEM images of stishovite crystals obtained at 350 °C (B) subducted oceanic slabs and crustal fragments in the Earth s man- and 450 °C (C) using glass starting material (D) close-up of Fig. 1C.(E) SEM tle (5). They are considered nominally anhydrous minerals (6), image of stishovite crystals obtained at 450 °C from coesite. although stishovite may contain small amounts of hydroxyl in con- junction with Al incorporation (7, 8). stishovite with about 11% coesite was obtained. Products at 400– There are large kinetic barriers associated with transforma- 550 °C were coesite-free stishovite. tions among silica polymorphs (9). Silica glass can be irreversibly Fig. 1 presents optical and scanning electron microscopy densified above 15 GPa without transformation to coesite or images for selected samples. Fig. 1A shows the polarized light stishovite (10, 11). Temperatures above approximately 1,000 °C micrograph of a partially transformed larger particle consisting of were required to constrain the coesite-stishovite equilibrium a coesite rim of about 20-μm thickness and a center of strained phase boundary (12). If kinetic barriers could be lowered, new, glass. The coesite rim shows undulatory extinction, suggesting it intermediate, high-pressure forms of silica may become accessi- is composed of oriented micrometer-sized domains that grow ble (13, 14). Additionally, low temperatures can afford nanostruc- μ tured forms of high-pressure silica phases (15). To explore routes inward from the surface. Stishovite occurs as fine, 0.5 to 1- m- for lowering kinetic barriers, we investigated a high-pressure sized, euhedral tabular crystals. In the 350 °C product from glass hydrothermal environment. Experiments at 10 GPa on silica starting material, stishovite crystals are peculiarly intergrown B glass–water or coesite–water mixtures at various temperatures (Fig. 1 ). The overall texture is most likely a result of the micro- (see SI Text for details) produced stishovite containing unprece- dented amounts of structural water. Author contributions: K.S., E.S., R.L.H., K.L., and G.P.H. performed research; K.S., J.N., E.S., R.L.H., K.L., G.P.H., and U.H. analyzed data; and A.N. and U.H. wrote the paper. Results and Discussion The authors declare no conflict of interest. The evolution of products during 8-h experiments using glass as 1To whom correspondence may be addressed. E-mail: [email protected] or starting material was as follows (Fig. S1). At 250 °C, the sample [email protected]. remained essentially amorphous; at 300 °C, coesite with about This article contains supporting information online at www.pnas.org/lookup/suppl/ 13% stishovite coexisted with some residual glass; and at 350 °C, doi:10.1073/pnas.1117152108/-/DCSupplemental. 20918–20922 ∣ PNAS ∣ December 27, 2011 ∣ vol. 108 ∣ no. 52 www.pnas.org/cgi/doi/10.1073/pnas.1117152108 Downloaded by guest on October 1, 2021 structure of coesite domains obtained initially from the hydro- The presence of H2O in stishovite in excess of 1 wt % is about thermal transformation of the glass. Samples prepared at higher three orders of magnitude higher than previously seen for Al-free temperatures consist of homogeneous stishovite crystals (Fig. 1C). stishovite and about one order of magnitude higher than ob- Previously intergrown crystals are now largely separated with the served for Al-bearing stishovite (7, 8). former intergrowth contacts clearly visible (Fig. 1D). In contrast, The differential scanning calorimetry (DSC) curves show when using coesite starting material, stishovite crystals appear strongly exothermic decomposition of stishovite to glass, consis- well sintered into agglomerates that have the shape and size of tent with previous thermodynamic studies (17). There are some the original coesite particles (Fig. 1E). We conclude that the differences in the shape and area of the peaks between the hydrothermal transformation of silica glass into stishovite pro- hydrous and dry samples, but the heat effects are not readily ceeds via coesite, and that the microstructure of coesite deter- quantified. A more accurate thermochemical approach utilizes mines the size and shape of stishovite crystals. high-temperature oxide melt drop solution calorimetry. Using The powder X-ray diffraction (PXRD) patterns of hydro- an appropriate thermochemical cycle (Table S2), one can calcu- thermally formed stishovite show that reflections are shifted late the enthalpy of the reaction, at ambient temperature: to lower Bragg angles (compared to dry stishovite, ref. 16), indi- ð Þþ ð Þ cating a slightly larger unit cell volume (Fig. 2A, Table 1, and SiO2 stishovite nH2O liquid c Table S1). Whereas the lattice parameter remains largely unaf- ¼ SiO2·nH2O ðhydrous stishoviteÞ: [1] fected, the a parameter increases by almost 0.5%. With increas- ing synthesis temperature, the a lattice parameter of anhydrous The calculated enthalpies of formation for samples 450-G and stishovite is approached. These changes, outside experimental 450-Co are 7.3 and 3.0 kJ∕mol, respectively. Assuming an error error, strongly suggest a structural role for H2O. of Æ0.2 wt % for the water content, the values above will change Secondary ion mass spectrometry (SIMS) was performed on by Æ0.4 kJ∕mol. The two materials have similar water contents the sample obtained from glass at 450 °C (i.e., sample 450-G, according to thermogravimetric analysis (TGA), but appear to see Table 1). Trace elements (B, Al, Na, Mg) did not exceed have somewhat different enthalpies. Differences between the two 60-wt-ppm and the H2O content was 1.3ð0.1Þ wt %. Selected hydrous stishovites became already apparent in the analysis of samples were subjected to thermal analysis (Fig. 2B and Table 1). their morphologies (see Fig. 1 D and E) and PXRD patterns Dry stishovite transforms exothermically into a glass at 550 °C. (see Fig. 2A). Further exploration of possible structural and The small weight loss below 300 °C in all samples represents energetic differences among differently prepared samples is the surface water. Hydrous stishovite 450-G decomposes at a lower subject of future studies. The salient point of the present findings temperature, 500 °C. Its decomposition is associated with a is that the incorporation of water into the stishovite has only a weight loss of 1.4 wt %, in agreement with the SIMS result, and small energetic penalty. Although the stishovite expands slightly we consider 1.3 Æ 0.2 wt % to be the water content of 450-G. with the incorporation of water (Table 1), the volume change for ACB TG (wt.%) DSC (mW/mg) exo 1.2 607 557 99.8 0.4 867 1.0 840 99.6 534 dry 99.4 0.2 0.8 583 99.2 550-G 0.6 99.0 0 Absorbance 0.4 450-Co 98.8 -0.2 98.6 0.2 450-G [1] 98.4 -0.4 0 EARTH, ATMOSPHERIC, AND PLANETARY SCIENCES 61.0 61.5 62.0 62.5 63.0 63.5 64.0 100 200 300 400 500 600 700 800 900 1200 1000 800 600 400 θ o o 2() T( C) Wavenumber (cm-1 ) F E D dry 0.60 1418 0.50 450-G 2653 0.40 2906 3389 dry bance 450-G 0.30 1422 Absor 0.20 3322 2889 2651 0.10 450-Co CP (450-G) 0 15 10 5 0 -170 -180 -190 -200 -210 4000 3500 3000 2500 2000 1500 1H Chemical Shift (ppm) 29Si Chemical Shift (ppm) Wavenumber (cm-1 ) Fig.
Details
-
File Typepdf
-
Upload Time-
-
Content LanguagesEnglish
-
Upload UserAnonymous/Not logged-in
-
File Pages5 Page
-
File Size-