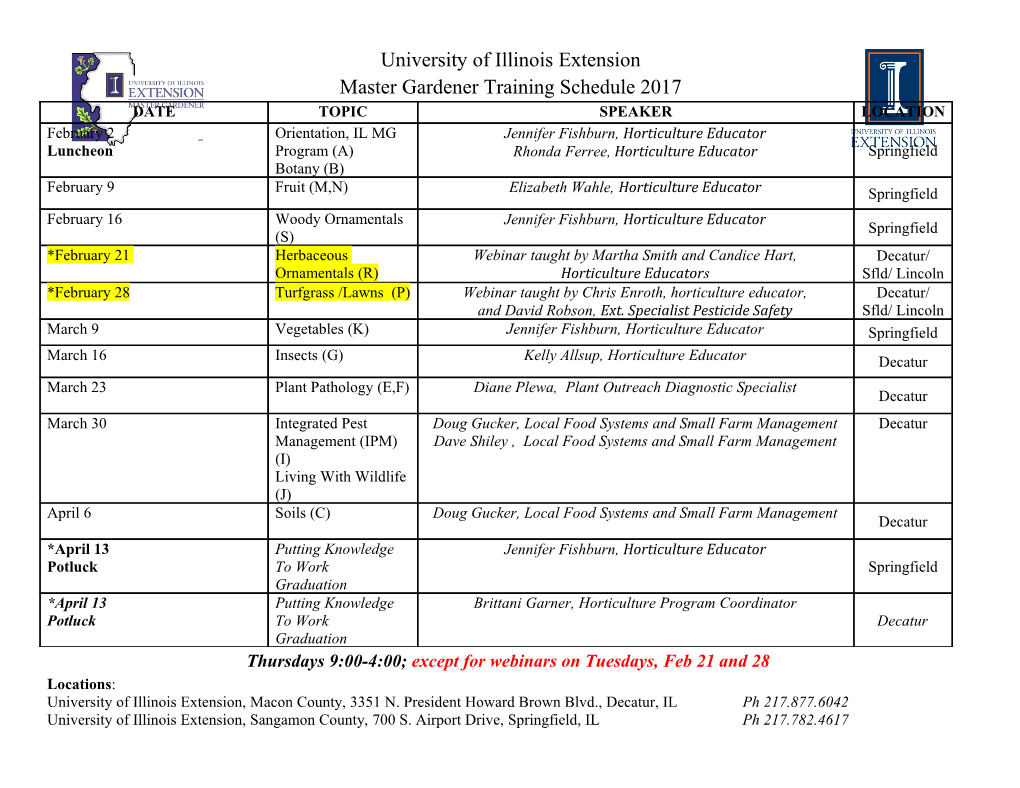
Nitroxyl and its anion in aqueous solutions: Spin states, protic equilibria, and reactivities toward oxygen and nitric oxide Vladimir Shafirovich*† and Sergei V. Lymar†‡ *Chemistry Department and Radiation and Solid-State Laboratory, New York University, New York, NY 10003; and ‡Chemistry Department, Brookhaven National Laboratory, Upton, NY 11973 Communicated by Norman Sutin, Brookhaven National Laboratory, Upton, NY, April 5, 2002 (received for review January 28, 2002) The thermodynamic properties of aqueous nitroxyl (HNO) and its However, nitrate, which is the peroxynitrite decomposition ؊ Ϫ anion (NO ) have been revised to show that the ground state of product, was not detected among the end products of HN2O3 NO؊ is triplet and that HNO in its singlet ground state has much decay (12). This result was interpreted as evidence against the -lower acidity, pKa(1HNO͞3NO؊) Ϸ 11.4, than previously believed. occurrence of reaction 2. On the other hand, the same research 2Ϫ These conclusions are in accord with the observed large differences ers reported peroxynitrite formation during N2O3 photolysis in ؊ 3 1 between HNO and NO in their reactivities toward O2 and NO. alkaline solution (13). To reconcile the data, it was suggested Laser flash photolysis was used to generate 1HNO and 3NO؊ by that thermal reaction 1 followed by deprotonation of HNO photochemical cleavage of trioxodinitrate (Angeli’s anion). The produces singlet NOϪ, which is the ground state in water, and ؊ 1 Ϫ 3 3 spin-allowed addition of O2 to NO produced peroxynitrite with that NO is unreactive toward O . In contrast, photochemical Ϫ 2 ؋ 9 ؊1⅐ ؊1 2 ؍ nearly diffusion-controlled rate (k 2.7 10 M s ). In contrast, cleavage of N2O3 was thought to generate the long-lived triplet 3 1 Ϫ the spin-forbidden addition of O2 to HNO was not detected (k ϽϽ excited state of NO , which reacted with O2. However, it seems ؋ 105 M؊1⅐s؊1). Both 1HNO and 3NO؊ reacted sequentially with unlikely that hydration can reverse a gas-phase energy gap of 3 ؊ ͞ 3 Ϫ two NO to generate N3O3 as a long-lived intermediate; the rate about 70 kJ mol between the ground state NO and the excited ؊ 1 Ϫ 1 laws of N3O3 formation were linear in concentrations of NO and state NO (10). Moreover, by analogy with O2, whose lifetime ؋ 109 in water is only 4 s (14), the existence of a long-lived excited 2.3 ؍ ؋ 106 M؊1⅐s؊1)orNOand3NO؊ (k 5.8 ؍ 1HNO (k Ϫ M؊1⅐s؊1). Catalysis by the hydroxide ion was observed for the state of NO also appears highly unlikely. Thus, reaction 2 1 remains obscure, particularly because others have reported O reactions of HNO with both O2 and NO. This effect is explicable by Ϫ 2 ,؋ 4 ؊1⅐ ؊1 consumption during spontaneous decomposition of HN O (2 ؍ ؊ a spin-forbidden deprotonation by OH (k 4.9 10 M s )of Ϫ 2 3 ؊ 3 1 the relatively unreactive HNO into the extremely reactive NO . 15) and detection of small amounts of NO3 upon completion of 1 Dimerization of HNO to produce N2O occurred much more slowly the reaction (16). ؋ 106 M؊1⅐s؊1) than previously suggested. The implications In the early 1970s two research groups used pulse radiolysis to 8 ؍ k) Ϫ of these results for evaluating the biological roles of nitroxyl are produce HNO͞NO by reaction between the hydrated electrons discussed. and NO (17, 18). This approach precluded examination of HNO͞NOϪ reactivities toward O because of a very rapid 2 Ϫ addition of two NO radicals to form N O , which then slowly itroxyl (HNO, also known as nitrosyl hydride) and its anion, 3 3 Ϫ decomposed to nitrous oxide and nitrite NNO , are the simplest molecules with nitrogen in the ϩ1 Ϫ Ϫ Ϫ oxidation state and yet their aqueous chemistry is not well NO ϩ 2NO 3 N O 3 N O ϩ NO . [3] understood. Recent suggestions that these redox neighbors of 3 3 2 2 the biologically important NO radical may play a role in cellular A pKa value of 4.7 for HNO was reported, although the spin metabolism (1–4) and in aerobic environments may be precur- states of NOϪ and HNO were not specified (17). The estimates Ϫ sors to cytotoxic peroxynitrite, ONOO , (5, 6) have engendered for the reduction potential of the NO͞NOϪ couple based on this Ϫ considerable interest in the chemistry of HNO͞NO . The value (19) are almost a volt higher than those obtained by characterization of these species is complicated by their insta- electrochemical techniques (20, 21); this discrepancy has never bility with respect to formation of nitrous oxide (7, 8). In most been addressed. Recent ab initio calculations placed the pKa of cases where nitroxyl has been invoked as an intermediate, the HNO at 7.2 (22). rate-determining step was its generation, a situation that allows In the hope of clarifying the HNO͞NOϪ chemistry, we have Ϫ little insight into the properties and reactivities of HNO͞NO used UV laser flash photolysis to produce HNO͞NOϪ species by Ϫ themselves. The NO anion is isoelectronic with O2 and, like O2, photochemical cleavage of Angeli’s anion and to investigate their should have a triplet ground state, whereas the ground state of reactivities. Here we present evidence that HNO has a much HNO should be a singlet. Indeed, these ground state assignments weaker acidity than previously believed. We show that the have been well established for HNO͞NOϪ in the gas phase deprotonation of HNO is a slow spin-forbidden process that (9, 10). controls the observed chemistry in alkaline solutions. The Ϫ Ϫ A frequently used source for aqueous HNO͞NO is trioxo- reactivities of HNO and NO toward O2 and NO have been 2Ϫ dinitrate (N2O3 , also known as Angeli’s anion), whose conju- investigated and a quantitative mechanistic description of these gate acid (H2N2O3) has consecutive pKa values of 2.5 and 9.7 reactions is presented. (11). It is widely accepted (7, 8) that slow decomposition of the monoprotonated anion occurs through heterolytic NON bond Materials and Methods cleavage Sample Solutions. All chemicals were of analytical grade and were used as received. Milli-Q purified water was used throughout. Ϫ 3 ϩ Ϫ HN2O3 HNO NO2 . [1] Stock solutions of Na2N2O3 (Cayman Chemical, Ann Arbor, MI) 2Ϫ in 10 mM NaOH were prepared daily. Relatively stable N2O3 Subsequent addition of O2 could yield peroxynitrite Ϫ Ϫ ͞ ϩ 3 ͞ † HNO NO O2 ONOOH ONOO . [2] To whom reprint requests should be addressed. E-mail: [email protected] or [email protected]. 7340–7345 ͉ PNAS ͉ May 28, 2002 ͉ vol. 99 ͉ no. 11 www.pnas.org͞cgi͞doi͞10.1073͞pnas.112202099 Downloaded by guest on September 23, 2021 0 1 ϭ ͞ ⅐ value and the tabulated entropy S ( HNOgas) 220.7 J (mol K) ⌬ 0 1 ϭ ͞ (27), we calculate fG ( HNOgas) 120 kJ mol. The free energy of HNO hydration is unknown, but is expected to be small by analogy with neutral molecules of similar dimensions and com- ⌬ 0 positions, e.g., HCN and H2CO, for which hydrG are approx- imately Ϫ5 and Ϫ1.7 kJ͞mol, respectively. The value ⌬ 0 1 Ϸ ͞ fG ( HNOaq) 115 kJ mol is, therefore, a reasonable estimate, which is also close to a 109 kJ͞mol value derived previously (19). The reduction potential of NO measured by the photoelec- trochemical technique has been reported as E0(NO͞NOϪ) ϭ Ϫ0.81 V vs. NHE without specifying the NOϪ spin state (21). This value is consistent with the upper limit E0(NO͞NOϪ) Ͻ Ϫ0.7 V vs. NHE that can be inferred from the onset of the irreversible NO reduction wave observed in controlled-potential coulometry (20). In both experiments the reducing electrons Fig. 1. Energy diagram for NO͞HNO͞NOϪ species in aqueous solution at 298 were supplied by the metal electrodes, a process for which there K and 1 mol͞kg standard states. Note the energy axis break at 120 kJ͞mol. The is no spin prohibition regardless of the product spin state. spectroscopic designations for the electronic states are given in parenthesis. Assuming, therefore, that these measurements pertain to the NO Ϫ Ϫ Ϫ 3 ⌬ 0 3 Ϸ ͞ Only the lowest excited states are shown for HNO and NO . reduction in the ground NO state, fG ( NOaq) 180 kJ mol ⌬ 0 3 Ϸ can be estimated. Finally, the values for fG ( HNOaq) 190 ͞ ⌬ 0 1 Ϫ Ϸ ͞ kJ mol and for fG ( NOaq) 248 kJ mol can be assigned under ϭ Ϫ1⅐ Ϫ1 [ 248 8,300 M cm (23)] sample solutions at pH 11–14.3 Ϫ a reasonable presumption that hydration does not appreciably 3 were prepared by diluting the Na2N2O3 stock. Unstable HN2O3 alter the gas-phase energy gaps of 75 kJ͞mol between HNO and ϭ Ϫ1⅐ Ϫ1 1 1 Ϫ 3 Ϫ [ 237 6,100 M cm (24)] sample solutions at pH 4–10 were HNO (9) and 68 kJ͞mol between NO and NO (10). 1 3 Ϫ prepared by flow-mixing equal volumes of the Na2N2O3 stock From the energy diagram, we estimate pKa( HNO͞ NO ) and 0.2 M phosphate, acetate, or borate buffers as described Ϸ11.4 and pKa(1HNO͞1NOϪ) Ϸ23, i.e., the acidity of HNO is below. Nitric oxide (Matheson) was purified by passing through very low. This result attests to the closer chemical similarity a scrubbing column with 2 M KOH and then through water. The between HNO and an aldehyde than between HNO and an ͞ ͞ various NO Ar and O2 Ar mixtures were produced by combin- oxyacid.
Details
-
File Typepdf
-
Upload Time-
-
Content LanguagesEnglish
-
Upload UserAnonymous/Not logged-in
-
File Pages6 Page
-
File Size-