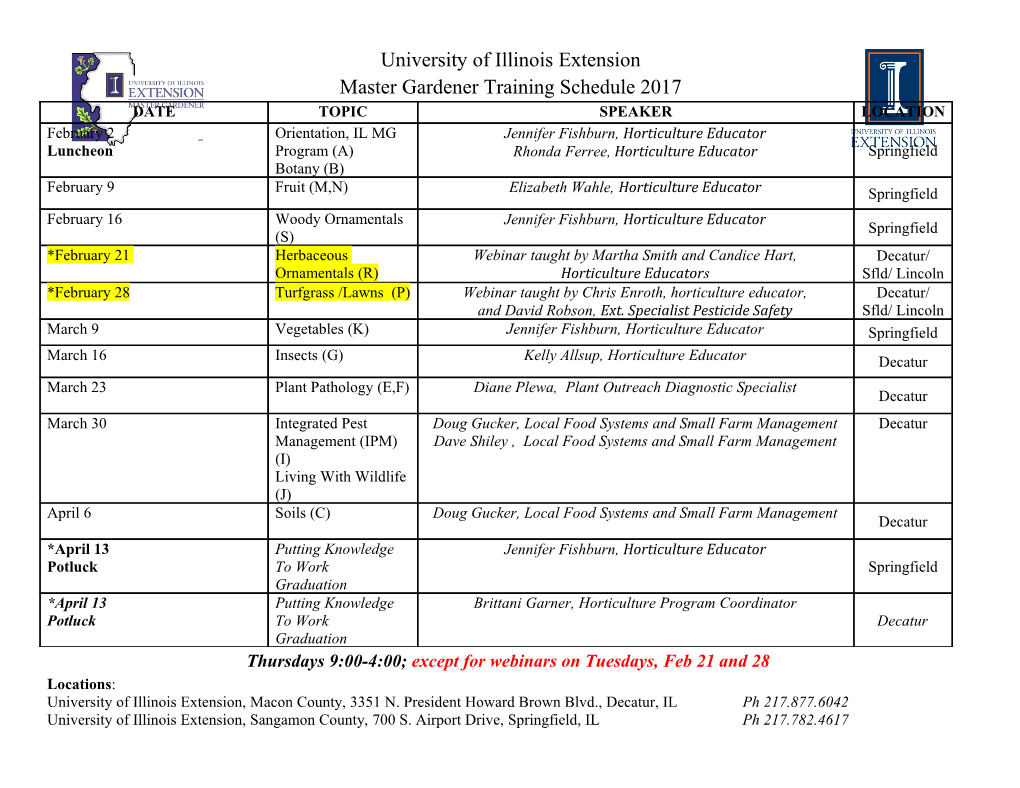
Superconducting Tunnel Junctions as Direct Detectors for Submillimeter Astronomy A Dissertation Presented to the Faculty of the Graduate School of Yale University in Candidacy for the Degree of Doctor of Philosophy by John Daniel Teufel Dissertation Director: Professor Robert J. Schoelkopf May 2008 UMI Number: 3317229 INFORMATION TO USERS The quality of this reproduction is dependent upon the quality of the copy submitted. Broken or indistinct print, colored or poor quality illustrations and photographs, print bleed-through, substandard margins, and improper alignment can adversely affect reproduction. In the unlikely event that the author did not send a complete manuscript and there are missing pages, these will be noted. Also, if unauthorized copyright material had to be removed, a note will indicate the deletion. ® UMI UMI Microform 3317229 Copyright 2008 by ProQuest LLC. All rights reserved. This microform edition is protected against unauthorized copying under Title 17, United States Code. ProQuest LLC 789 E. Eisenhower Parkway PO Box 1346 Ann Arbor, Ml 48106-1346 ©2008 by John Daniel Teufel. All rights reserved. Abstract Superconducting Tunnel Junctions as Direct Detectors for Submillimeter Astronomy John Daniel Teufel 2008 This thesis presents measurements on the of performance of superconducting tunnel junctions (STJ) as direct detectors for submillimeter radiation. Over the past several decades, STJ's have been successfully implemented as energy-resolving detectors of X-ray and optical photons. This work extends their application to ultra-sensitive direct detection of photons near 100 GHz. The focus of this research is to integrate the detector with a readout that is sensitive, fast, and able to be scaled for use in large format arrays. We demonstrate the performance of a radio frequency single electron transistor (RF-SET) configured as a transimpedance current amplifier as one such readout. Unlike traditional semiconductor amplifiers, the RF-SET is compatible with cryogenic operation and naturally lends itself to frequency domain multiplexing. This research progressed to the invention of RF-STJ, whereby the same RF refiectometry as used in the RF-SET is applied directly to the detector junction. This results in a greatly simplified design that preserves many of the advantages of the RF-SET while achieving comparable sensitivity. These experiments culminate in calibration of the detector with an on-chip, mesoscopic noise source. Millimeter wave Johnson noise from a gold microbridge illuminates the detector in situ. This allows for direct measurement of the "optical" properties of the detector and its RF readout, including the response time, responsivity and sensitivity. Acknowledgements I would like to take these few paragraphs to recognize some of the people who were instrumental in both the completion of this thesis as well as my progress as a scientist. To begin, I would like to thank my parents for always encouraging my love of science. This includes my father teaching me the importance of asking the right questions and my mother making sure I worked hard at whatever it was I decided to pursue. While I learned most of the facts and equations as an undergraduate, it was as a graduate student at Yale that I learned what it means to be a physicist. Most importantly I would like to thank my advisor, Rob Schoelkopf. His example has had a great influence on how I think about physics, the way in which I approach an experiment, and how to debug it when things are not working as they should. Never underestimate a man who can smell a ground loop from ten feet away. Dan Prober has served as my second advisor. I appreciate his open door and willingness to discuss the details of a past, present or future experiment. This includes both tracking down the last factor of two in an equation and providing perspective of where our daily research fits into the bigger picture. I have also learned a great deal from Michel Devoret. I am constantly rediscovering all the levels in which his simple, yet precise analogies, aptly explain even the most complicated physics. In general, it has been a pleasure to work on the 4th floor of Becton Center; the Schoelkopf, Prober, and Devoret labs demonstrate that innovative research is the natural byproduct of an active and inspiring scientific environment. This is the direct result of the many postdocs and graduate students in these labs. Specifically, I want to thank Luigi Frunzio, Bertrand Reulet, Etienne Boaknin, Irfan Siddiqi, Chris Wilson, Andreas Wallraff, Hannes Majer, and Andrew Houck. Just as important, I am indebted to my fellow graduate students with whom I overlapped to most: Lafe Spietz, Minghao Shen, Ben Turek, Dave Schuster, Julie Love, Matt Reese, Vijay, and Veronica Savu. I especially need to thank Konrad Lehnert for his role both at the start and finish of this work. It was his example that shaped much of who I am as an experimentalist; and his support that allowed me to see it through to fruition. Lastly, I want to thank my wife, Rachael. She has been nothing but supportive and understanding over the entire process of my graduate education. I would not be where I am, or who I am, today without her. 1 Contents 1 Introduction 13 1.1 Overall Motivation 14 1.2 General Operating Principle for the STJ 17 1.3 Readout Techniques 21 1.4 Thesis Overview 21 2 Theory of Superconducting Tunnel Junctions 23 2.1 Introduction to BCS Theory 23 2.2 Josephson Tunneling Currents 25 2.3 Quasiparticle Tunneling Currents 27 2.4 Other Tunnel Junction Physics 29 2.5 Complex Conductivity of a Superconductor 31 2.6 Quasiparticle Dynamics 34 3 Radio Frequency Techniques 36 3.1 Impedance and Reflection 38 3.2 Bode-Fano Limit 40 3.3 The L Section Transformer 41 3.4 Practical Considerations 45 3.5 Multistage Transformers 47 3.6 Frequency Domain Multiplexing 51 3.7 Noise and Sensitivity 52 4 Device Fabrication 58 4.1 Overview of E-Beam Lithography 58 4.2 Tunnel Junction Fabrication at Yale 60 4.3 Junction Properties 60 4.4 Design Considerations 63 2 CONTENTS 3 5 Experimental Setup 65 5.1 General Filtering Requirements 65 5.2 Cryostat Overview 70 5.3 Sample Holder 74 5.4 Cables and Wiring 74 5.5 RF Measurement Setup 76 5.6 Cryogenic Amplifiers 78 5.7 DC Measurement Setup 79 6 DC Characterization of Tunnel Junctions 81 6.1 General Characteristics of an SIS Tunnel Junction 82 6.2 Cooper Pair Tunnelling Currents 83 6.3 Quasiparticle Currents 86 6.4 Conclusion 90 7 Single Electron Transistors as Current Amplifiers 92 7.1 Current Amplifier Technology 94 7.2 SET Physics 95 7.3 SET Design and Layout 99 7.4 DC Characterization of SETs 101 7.5 RF-SET Charactrerization 104 7.6 RF-SET Configured as a Transimpedance Current Amplifier 115 8 Radio Frequency Superconducting Tunnel Junction 125 8.1 RF-STJ: Readout Concept 126 8.2 Advantages of Direct RF Readout 127 8.3 Impedance Matching to Subgap Currents 128 8.4 Noise and Sensitivity 130 8.5 Characterization of RF Readout 133 8.6 Characterization of Sensitivity 136 8.7 Readout Timescales 140 8.8 Conclusions 142 9 Submillimeter Photon Sources 143 9.1 Requirements for Photon Sources 144 9.2 Cryogenic Blackbody as a Calibration Source 145 9.3 Quasi-Optically Coupling to a Macroscopic Bolometer 146 9.4 Mesoscopic Resistor as a Photon Source 151 CONTENTS 4 9.4.1 Hot Electron Regime 153 9.4.2 Non-interacting Electron Regime 155 9.4.3 Comparison of the Two Regimes 157 9.5 Design and Layout of Gold Microbridge 159 9.6 Calibration of Microbridge Noise at RF Frequencies 160 9.7 Conclusions 162 10 Detector Characterization with Photon Source 163 10.1 Design Principles 164 10.2 Fabrication 167 10.3 Detector Characterization 168 10.4 RF Characterization 170 10.5 Dilution Refrigerator Measurements 174 10.6 Redesign of Chip Layout 177 10.7 Measurements of Redesigned Source and Detectors 181 10.8 Conclusions 188 11 Conclusions 191 11.1 Review of Conclusions Drawn from this Work 191 11.2 Relevance of this Work to the Detector Community 194 11.3 Possible Future Applications 195 References 196 List of Figures 1.1 Raleigh-Jeans vs Wien limit 14 1.2 Detector classifications 16 1.3 Antenna-coupled STJ 18 1.4 Quasiparticle creation and confinement 19 2.1 Quasiparticle density in aluminum 24 2.2 Critical current modulation for a SQUID 27 2.3 Subgap current predicted by BCS theory 28 2.4 Mattis-Bardeen theory for the complex impedance of a superconductor 32 2.5 Quasiparticle recombination time in aluminum 35 3.1 Bode-Fano limit 39 3.2 DC matching circuit 40 3.3 L section matching network for high impedances 42 3.4 Lorentzian approximation for a tank circuit 43 3.5 Tank circuit bandwidth 44 3.6 L section matching network for low impedances 45 3.7 Inductor self-resonance 46 3.8 Two-stage transformer for maximal bandwidth 48 3.9 Two stage transformer for maximal impedance match 49 3.10 Frequency domain multiplexing 51 3.11 Noise temperature of an amplifier chain 53 3.12 Effective noise temperature of a resonantly coupled amplifier 56 4.1 Dolan bridge technique 59 4.2 SQUID SEM 61 4.3 Tilted SEM image of a tunnel junction 62 5.1 Filtering requirements 66 5 LIST OF FIGURES 6 5.2 General RC lowpass filter characteristics 67 5.3 Tapeworm filter assembly 68 5.4 Tapeworm filter integration with cryostat 69 5.5 Bode plots of three types of lowpass filters 70 5.6 Heliox 3He Cryostat 71 5.7 Heliox operating principle 72 5.8 Sample Holder 73 5.9 Measurement setup 75 5.10 RF measurement configuration 77 5.11 Amplifier characteristics 78 5.12 HEMT gain and noise temperature 79 5.13 DC measurement configuration
Details
-
File Typepdf
-
Upload Time-
-
Content LanguagesEnglish
-
Upload UserAnonymous/Not logged-in
-
File Pages211 Page
-
File Size-