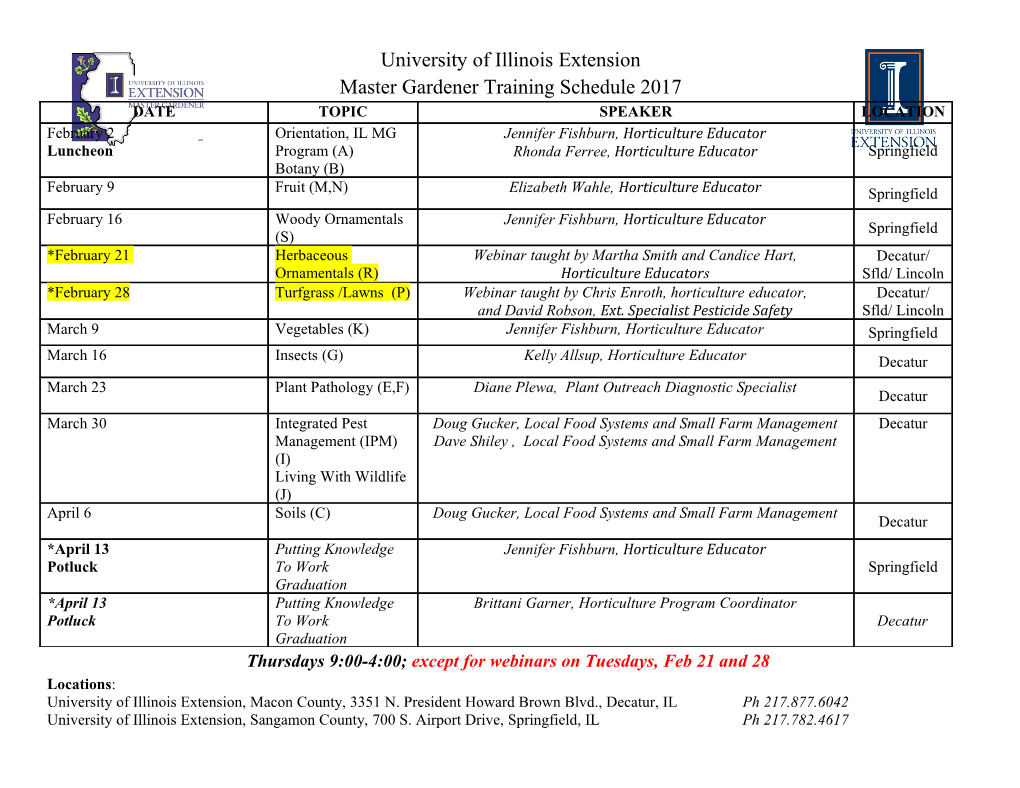
Interfaces in amorphous/crystalline silicon heterojunction solar cells vorgelegt von M. Sc. Mathias Mews geboren in Gera von der Fakultät IV - Elektrotechnik und Informatik der Technischen Universität Berlin zur Erlangung des akademischen Grades Doktor der Naturwissenschaften - Dr. rer. nat. - genehmigte Dissertation Promotionsausschuss: Vorsitzender: Prof. Dr. Christian Boit Gutachter: Prof. Dr. Bernd Rech Prof. Dr. Pere Roca i Cabarrocas Prof. Dr. Bernd Szyszka Tag der wissenschaftlichen Aussprache: 30.Mai 2016 Berlin 2016 Contents 1 Introduction 1 2 Materials, interfaces and devices 4 2.1 Amorphous silicon . 4 2.1.1 Hydrogen in amorphous silicon . 4 2.1.2 Amorphous silicon density of states . 5 2.1.3 Doping in amorphous silicon . 7 2.2 Amorphous/crystalline silicon heterojunctions . 7 2.2.1 Amorphous/crystalline silicon heterojunction solar cells . 7 2.2.2 Interface properties . 10 2.3 Recombination . 12 2.4 Solar cells . 13 3 Preparation, spectroscopy and simulation 15 3.1 Sample preparation . 15 3.1.1 Wafer pre-treatment . 15 3.1.2 Plasma-enhanced chemical vapor deposition . 15 3.1.3 Hydrogen plasma treatments of a-Si:H . 17 3.1.4 Liquid silicon precursors . 18 3.1.5 Black silicon . 18 3.1.6 Solar cell fabrication . 21 3.2 Spectroscopy and device simulation . 21 3.2.1 Transient photoconductive decay measurements . 21 3.2.2 Fourier transform infrared spectroscopy . 23 3.2.3 Spectral ellipsometry . 24 3.2.4 Photoelectron spectroscopy . 25 3.2.5 Surface photovoltage . 28 3.2.6 Auxiliary measurement methods . 29 3.2.7 Device simulation . 30 i 4 Hydrogen plasma treatments 31 4.1 Abstract . 31 4.2 Introduction . 32 4.3 Experimental details . 33 4.4 Hydrogen plasma treatments of a-Si:H . 34 4.4.1 Carrier lifetime spectroscopy . 34 4.4.2 Diffusion profiles . 36 4.4.3 Hydrogen density, mass density and band gap . 38 4.4.4 Valence band spectroscopy . 40 4.4.5 Solar cell results . 41 4.5 Conclusion . 43 5 Black silicon texture 44 5.1 Abstract . 44 5.2 Nanotextures and (i)a-Si:H passivation . 45 5.3 Introduction . 47 5.4 Experimental details . 47 5.5 Results and discussion . 48 5.5.1 Reflectivity of black silicon surfaces and solar cells . 48 5.5.2 Passivation layer optimization . 50 5.5.3 Quantum efficiency measurements . 53 5.5.4 Parasitic absorption in black silicon nanostructures . 55 5.6 Conclusion . 55 6 Solution-processed amorphous silicon 58 6.1 Abstract . 58 6.2 Promise and challenge of liquid silicon . 59 6.3 Introduction . 60 6.4 Experimental details . 61 6.5 Results and Discussion . 62 6.5.1 From polysilane to amorphous silicon . 62 6.5.2 Valence band spectroscopy . 64 6.5.3 Liquid processed amorphous silicon passivation layers . 65 6.5.4 Oxidation of liquid processed amorphous silicon . 67 6.6 Conclusion . 69 7 Valence band alignment and hole transport 70 7.1 Abstract . 70 ii 7.2 SHJ valence band offset modification . 71 7.3 Introduction . 73 7.4 Experimental and simulation details . 75 7.5 Results and discussion . 76 7.5.1 SHJ valence band alignment and passivation . 76 7.5.2 Valence band offset and transport across the SHJ . 77 7.5.3 Valence band stairwell . 79 7.6 Conclusion . 80 8 Prospects for silicon heterojunction solar cells 83 8.1 Introduction . 83 8.2 Carrier selective contacts in SHJ solar cells . 84 8.2.1 Charge selective contacts . 84 8.2.2 ITO/(p)a-Si:H contact . 86 8.2.3 Hole contact materials . 87 8.2.4 Oxygen vacancies in tungsten oxide hole collection layers . 89 8.2.5 Electron contact materials . 92 8.3 Back-contact back-junction solar cells . 94 8.3.1 state of the art . 94 8.3.2 Printable silicon wafer based BC-BJ solar cells . 95 8.4 Crystalline silicon based tandem solar cells . 96 8.4.1 General concept . 96 8.4.2 Tandems with perovskites . 97 8.4.3 Tandems with InGaP and related materials . 99 8.4.4 Summary of crystalline silicon based tandem concepts . 99 8.5 Conclusion . 100 9 Conclusions and outlook 101 10 Appendix 104 10.1 Abbreviations and symbols . 104 10.2 Publications . 107 10.3 Acknowledgments . 111 iii Chapter 1 Introduction The first monocrystalline silicon solar cells with a p/n-junction were fabricated in 1954 [1]. Today more than 200 GW photovoltaic capacity are installed worldwide and the annual production capacity is about 40 GW [2]. 92 % of the global PV produc- tion and therefore most of the installed capacity are crystalline silicon based modules, with multi-crystalline silicon contributing 56 % and mono-crystalline silicon contribut- ing 46 % of the overall market [3]. The first solar cells fabricated at Bell Labs only had an efficiency of about 6 % and featured a diffused p/n-junction [1] This technol- ogy gradually evolved by etching random pyramid structures on the wafer to suppress reflection [4], adding a front surface anti-reflection layer [5] and emitter passivation layer [6] and by applying locally diffused rear contacts to limit recombination [7], fi- nally achieving 25 % efficiency in 1998 [8]. One feature of this technology is the direct contact of the diffused emitter and base contacts to the metal contacts, which limits the achievable output voltage of silicon solar cells with diffused junctions [8]. A different crystalline silicon based approach was presented by Sanyo in 1992 [9]. In- stead of relying on a diffused p/n-junction the amorphous/crystalline silicon hetero- junction (SHJ) uses doped and hydrogenated amorphous silicon (a-Si:H) layers to form the p/n-junction and the base contact. The use of doped a-Si:H layers instead of dif- fused dopants offers two advantages. First a passivation layer can be inserted into the junction. In the case of the SHJ this is intrinsic hydrogenated amorphous silicon ((i)a-Si:H), which reduces the defect density in the junction and enables SHJ solar cells to reach open circuit voltages of up to 750 mV [10], in contrast to diffused silicon homojunctions, which are limited to about 700 mV [8]. Secondly a-Si:H depositions are conducted at roughly 200 ◦C, in contrast to diffused junction formation at about 1000 ◦C. The SHJ technology offers higher efficiencies [11], better temperature coeffi- cients [12] and lower life-cycle greenhouse gas emissions [13] than the the more wide spread silicon homojunction technology. Also, the fabrication cost per peak power gen- 1 2 CHAPTER 1. INTRODUCTION eration of both technologies is comparable [14]. The development of SHJ solar cells was driven by record efficiencies from Sanyo (Pana- sonic) [9, 10, 15–18] with the latest record power conversion efficiency of 25.6 % [11]. Studies published by research institutes found that the amorphous/crystalline silicon interface needs to be devoid of epitaxial growth [19, 20] and that high hydrogen con- centrations at the interface are beneficial [21]. Furthermore it is necessary to add a transparent conductive oxide (TCO) on the doped a-Si:H layers, since their conductiv- ity is not sufficient for lateral carrier transport towards the metal grid fingers [22]. The interface between the commonly used TCO indium tin oxide and the p-doped a-Si:H is a tunnel-recombination junction [23] with non-ideal band alignment, which leads to inherent limitations of the fill factor [24, 25]. One disadvantage of SHJ solar cells is parasitic absorption in a-Si:H and TCO layers [26], which triggered work on rear- emitter [27] and back-contact back-junction [28, 29] devices, to decrease the parasitic absorption. The work presented in this thesis relates to many of this points. Chapter 4 discusses a process designed to fabricate epitaxial free (i)a-Si:H passivation layers. Chapter 5 deals with the application of "black" silicon [30] as anti-reflection structure for SHJ solar cells. Chapter 6 discusses the application of liquid silicon precursors [31] for SHJ solar cells. Chapter 7 discusses the band alignment at the SHJ and its influence on hole transport. Chapter 8 discusses alternative contact schemes to overcome the fill factor limitations of SHJ solar cells and potential applications of SHJ in tandem devices [32] to overcome the current record of 25.6 % power conversion efficiency. Structure of this thesis This thesis contains two chapters, with short summaries of the necessary basics to understand the later experimental results, four chapters detailing these results and a chapter discussing the status and future prospects of the SHJ technology. The four results chapters each are based on an article published in a peer-reviewed scientific journal. Two chapters include additional results, which were not part of the original publication. Chapter 2 gives a brief summary of the materials and device concepts relevant to this thesis and references to more detailed literature. Chapter 3 gives descriptions of the preparation, spectroscopy and device simulation techniques used in this thesis. Chapter 4 is based on a publication about hydrogen plasma treatments of SHJs [33]. A paragraph about related solar cell results is added to the already published study. Chapter 5 details a study on nanotextured substrates for use in SHJ solar cells [34]. 3 The chapter starts with a short description of the changes in the approach presented in the preceding chapter and how they relate to this study and is followed by the expanded reprint of the actual study. Chapter 6 deals with the liquid silicon precursor neopentasilane and its application for the preparation of a-Si:H passivation layers [35]. Chapter 7 discusses the relation between the valence band offset at the SHJ and the solar cell transport properties [36].
Details
-
File Typepdf
-
Upload Time-
-
Content LanguagesEnglish
-
Upload UserAnonymous/Not logged-in
-
File Pages135 Page
-
File Size-