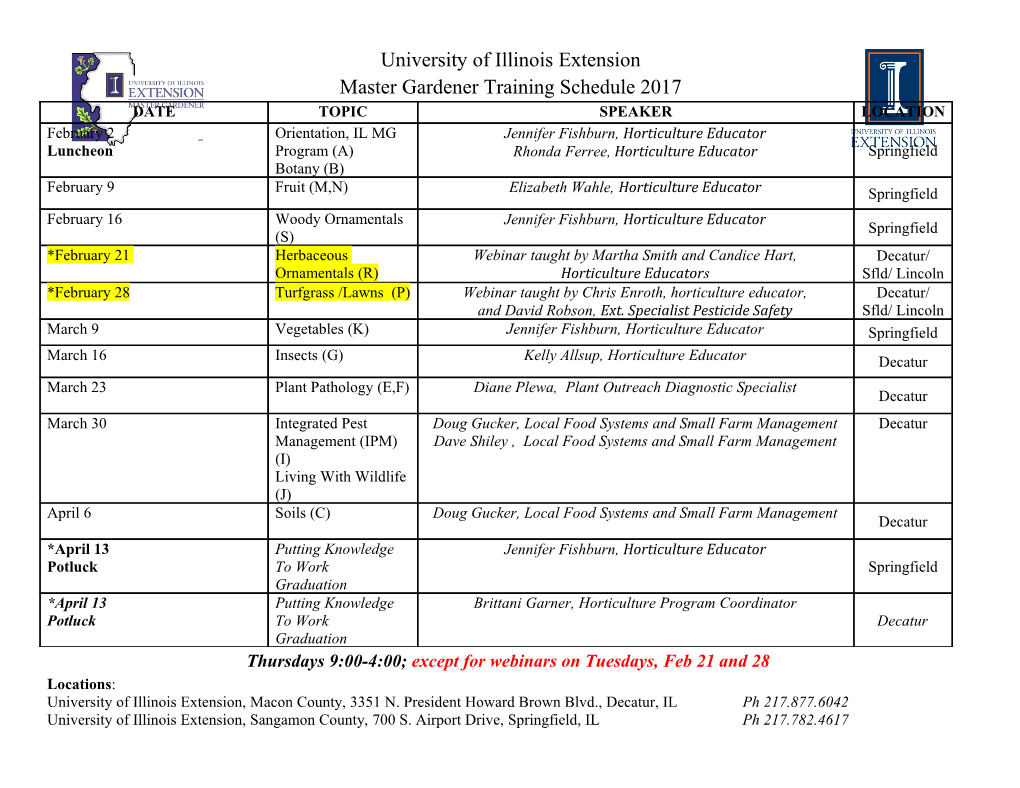
RMo3A-4 A 180-GHz Super-Regenerative Oscillator with up to 58 dB Gain for Efficient Phase Recovery Hatem Ghaleb#, Christian Carlowitz$, David Fritsche #, Corrado Carta#, and Frank Ellinger# #Chair for Circuit Design and Network Theory, Technische Universität Dresden, Germany $Institute of Microwaves and Photonics, University of Erlangen-Nuremberg, Germany Abstract—This paper reports on the design of a 180-GHz super-regenerative oscillator in a 130nm SiGe BiCMOS VCC 0° OUT technology. The oscillator has a tuning range of 6.5%, an output 180° TL TL TL power of 0.5 dBm, and occupies an area of 0.72 mm2. When sh TLcc cc sh Balun/ Combiner operated with a periodic quench signal, the circuit requires a TL Ct Ct TLsr minimum input power of -58 dBm for a phase coherent output, sr TLad TLad and can be switched at a rate up to 10 GHz. The circuit has a dc Oscillator power consumption of 8.8 mW, and a maximum regenerative Core T R T gain of 58 dB. QPSK and 8-PSK modulation up to 3 Gbit/s have 3 cas 4 been demonstrated at an energy efficiency of 2.9 pJ/bit. To the Vcas best knowledge of the authors, this work is the fastest reported TLres TLres T1 T2 phase-sampling super-regenerative oscillator to date. Rb Keywords— 200 GHz band, super-regenerative receiver. Vb TLee T5 T6 TLee Iref I. INTRODUCTION Rt The rising demand for high data rates in mobile wireless Vtune communication raises interest in the millimeter-wave frequency band (30-300 GHz) due to the availability of large T7 T8 T11 and unallocated contiguous bandwidth. This enables high- TLin L1 speed communication applications at low or moderate spectral INJ SW T12 M2 M1 efficiency. However, it comes at the cost of high power T10 consumption, as the transistor gain decreases and multiple T9 Input Stage Quenching cascaded amplifier stages are needed. Thus, a transceiver architecture based on the use of a Fig. 1 Schematic of the 180-GHz SRO super-regenerative oscillator (SRO) with phase and amplitude sampling capability has been proposed [1] and demonstrated This limitation is, however, relieved by the high bandwidth at 5.8 GHz [2]. The concept relies on the use of positive in mm-wave bands, as well as the high path loss, which allows feedback in the oscillator to generate a very large regenerative for frequency reuse after a short distance. In this paper, the gain [3]. The information signal is fed to the oscillator core design and measurements of a 180-GHz SRO IC are presented. and the phase is sampled at the turn-on instant, when the oscillator is most sensitive. The oscillation then grows II. CIRCUIT DESIGN AND LAYOUT exponentially to its steady-state level with the phase Fig. 1 shows a schematic diagram of the SRO circuit, information preserved. To receive the following symbols, the including all input and output signals. The oscillator core is oscillator has to be periodically quenched, thus each realized with the transistors T1-4, with the diode-connected oscillation pulse represents one symbol. Additionally, by transistor pair T5,6 used as varactors for oscillation frequency quenching the oscillator before a steady state is reached, the tuning through the tuning voltage Vtune. amplitude of the input signal can also be regenerated, leading The core is based on the differential common-collector to the use of the SRO with amplitude modulation, phase Colpitts topology, which has demonstrated superior modulation, or both. performance at mm-wave frequencies [4]. A differential The advantages of this super-regenerative approach common-base output buffer consisting of transistors T3,4 include low complexity and high power and area efficiency, boosts the output power delivered to the load, and ensures since one block can replace multiple gain stages in the sufficient reverse isolation to prevent the locking of the receiver. Through pulsed operation by repeated quenching, the oscillator to reflected pulses, in case of output impedance power consumption is further reduced. On the other hand, the mismatch. The input transconductance stage consisting of high sensitivity of the super-regenerative circuit leads to transistors T7-10 acts as an active balun, injecting differential susceptibility to interferers in adjacent channels. input currents into the oscillator. 978-1-7281-1701-0/19/$31.00 © 2019 IEEE 131 2019 IEEE Radio Frequency Integrated Circuits Symposium 188 3 186 2 184 1 182 0 (dBm) (GHz) 180 -1 out osc P f 178 -2 Oscillation frequency 176 -3 Output power 174 -4 1.0 1.5 2.0 2.5 3.0 3.5 4.0 4.5 5.0 Vtune (V) Fig. 3 fosc and Pout vs Vtune from measurements (solid) and simulations (dashed) The dc routing is done using multi-layer high-capacitance zero-Ohm lines [6]. The circuit is fabricated in a 130nm SiGe Fig. 2 Micrograph of the SRO chip with pad labels (area = 900 800 m2) BiCMOS HBT technology with fT/fmax up to 300/450 GHz. Additionally, it helps to provide a broadband match due to The total chip area including the pads is 0.72 mm2. A labeled the low resistive input impedance, which is transformed to micrograph of the SRO IC is shown in Fig. 2. 50 by the short transmission line segment TLin as well as the pad capacitance. A small inductor L1 adjusts the phase balance III. MEASUREMENT RESULTS between the two balun branches. The SRO circuit was measured on-wafer with the dc and The diode-connected transistors T11,12 form a current the SW pads wire-bonded on a custom PCB. The dc bias was mirror with T7,9,10, which allows the current biasing of the set at VCC = 4.4 V, Vcas = 4.1 V and Vb = 3.2 V. The circuit oscillator through Iref. T8 maintains the voltage common-mode draws 6 mA during free-running operation. A Virginia Diodes balance between the two differential branches. By adding the PM4 power meter was used for signal power measurements NMOS transistor M1, a signal SW can be applied to and the calibration of measurement setup losses. For the periodically quench the oscillator as required, by sinking Iref spectrum measurements, a Radiometer Physics HM140-220 and pulling down the bias voltage node. harmonic mixer was used in combination with a By adding capacitance to that node, the quenching slope of Rohde&Schwarz FSW67 spectrum analyzer. The CW input the oscillator at the turn-on instant can be slowed. This has signal was generated using a Rohde&Schwarz ZVA-Z220 been found to improve the SRO sensitivity in terms of the network analyzer converter. minimum input power required for low-BER phase recovery Fig. 3 shows the measured oscillation frequency fosc and Pinj,min [5]. However, a large capacitance would limit the the output power Pout as functions of Vtune, with both INJ and quench rate, thus reducing the achievable symbol rate. SW inputs terminated to 50 . The frequency tuning range Therefore, an optimum value for the capacitance has been extends between 175.9187.7 GHz, which amounts to 6.5%, investigated and implemented with the NMOS capacitor M2. or almost 12 GHz. The output power at 180 GHz is -2 dBm, The supply voltage VCC is provided through the feed lines whereas the maximum output power is 0.5 dBm. TLcc. The transmission lines TLad, TLsh and TLsr, as well as the capacitors Ct form an impedance transformation network at a) -20 b) -20 -30 -30 the output. The optimum load impedance was investigated by -40 -40 load-pull simulations, which used fast start-up rather than the -50 -50 P (dBm) P (dBm) P highest output power as the optimization goal. A high -60 -60 inductive part was found to be desirable, whereas a high -70 -70 -80 -80 170 175 180 185 190 170 175 180 185 190 resistive part led to a higher steady-state swing, but also a f (GHz) f (GHz) higher start-up time constant. Therefore, an optimum value Fig. 4 Measured SRO spectrum for f = 180 GHz, f = 1 GHz, and was chosen for the load resistance as a tradeoff between the inj sw (a) Pinj = -58 dBm, (b) Pinj = -48 dBm start-up time and the steady-state output power. a) -20 b) -20 All transmission lines were implemented as grounded -30 -30 coplanar waveguides (GCPW), and all bipolar transistors were -40 -40 2 -50 -50 P (dBm) P (dBm) P sized at the emitter area AE = 2 0.9 0.07 m . The -60 -60 differential outputs are combined in a passive microstrip balun, -70 -70 -80 -80 which consists of one /4 and one 3/4 transmission line 160 165 170 175 180 185 190 195 200 150 160 170 180 190 200 210 segments, both with a characteristic impedance of 71 . The f (GHz) f (GHz) balun also performs the function of impedance transformation Fig. 5 Measured SRO spectrum for finj = 180 GHz, Pinj = -26 dBm, and of single-ended 50 to differential 100 . (a) fsw = 5 GHz, (b) fsw = 10 GHz 132 Fig. 7 Measured IF (top) and complex baseband (bottom) signals in linear and logarithmic mode, showing the regenerated communication signal Fig. 6 Measurement setup for demonstrating SRO-based regeneration of If this occurs before a steady-state amplitude is reached, n-PSK modulated signals the SRO is said to be operating in linear mode, due to the linear dependence between input and output amplitudes. To test the regenerative sampling capability of RF and Otherwise, it is said to be in logarithmic mode.
Details
-
File Typepdf
-
Upload Time-
-
Content LanguagesEnglish
-
Upload UserAnonymous/Not logged-in
-
File Pages4 Page
-
File Size-