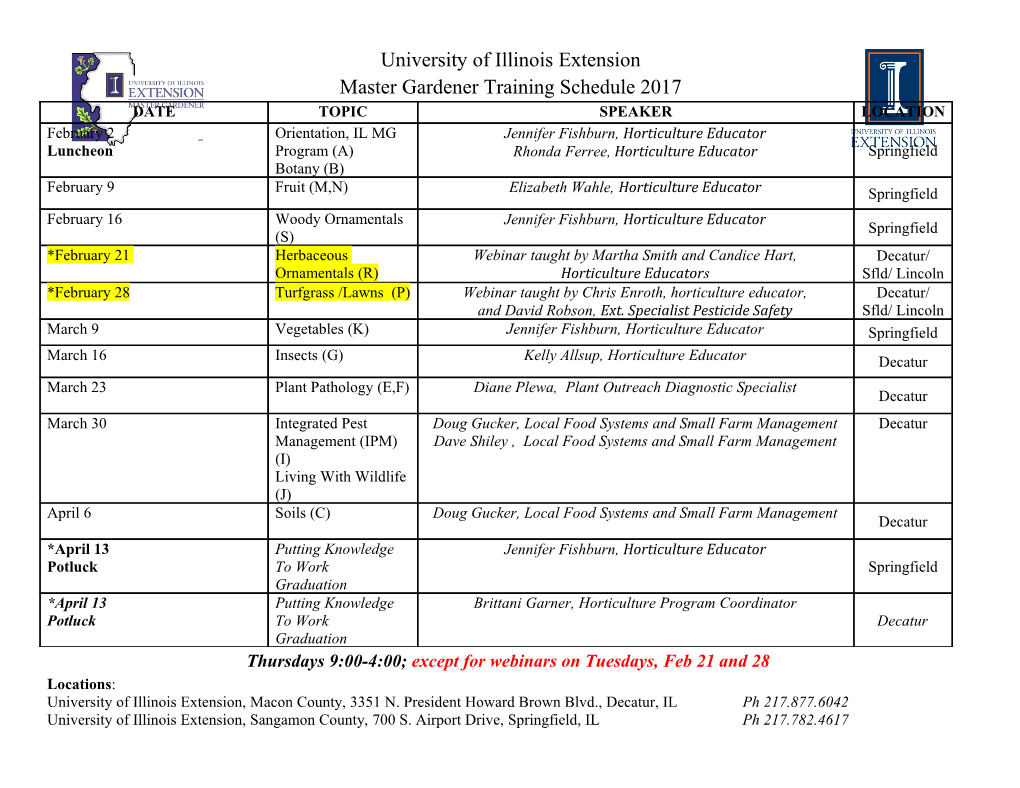
RICE UNIVERSITY Manipulation of Electromagnetic Fields with Plasmonic Nanostructures: Nonlinear Frequency Mixing, Optical Manipulation, Enhancement and Suppression of Photocurrent in a Silicon Photodiode, and Surface-Enhanced Spectroscopy by Nathaniel K Grady A THESIS SUBMITTED IN PARTIAL FULFILLMENT OF THE REQUIREMENTS FOR THE DEGREE Doctor of Philosophy APPROVED, THESIS COMMITTEE: Jaom^JTHalas, Stanley C. Moore Professor of Electrical and Computer Engineering, Professor of Bioengineering, Professor of Chemistry, Professor of Physics Astronomy, Chair Peter Nordlander, Professor of Physics and Astronomy, Professor of Electrical and Computer Engineering Stephan Innk, Assistant Professor of Chemistry, Assistant Professor of Electrical and Computer Engineering HOUSTON, TX MAY 2010 UMI Number: 3421182 All rights reserved INFORMATION TO ALL USERS The quality of this reproduction is dependent upon the quality of the copy submitted. In the unlikely event that the author did not send a complete manuscript and there are missing pages, these will be noted. Also, if material had to be removed, a note will indicate the deletion. Dissertation Publishing UMI 3421182 Copyright 2010 by ProQuest LLC. All rights reserved. This edition of the work is protected against unauthorized copying under Title 17, United States Code. ProQuest LLC 789 East Eisenhower Parkway P.O. Box 1346 Ann Arbor, Ml 48106-1346 ABSTRACT Manipulation of Electromagnetic Fields with Plasmonic Nanostructures: Nonlinear Frequency Mixing, Optical Manipulation, Enhancement and Suppression of Photocurrent in a Silicon Photodiode, and Surface-Enhanced Spectroscopy by Nathaniel K Grady Metallic nanostructures are one of the most versatile tools available for manipulating light at the nanoscale. These nanostructures support surface plasmons, which are collective excitations of the conduction electrons that can exist as propagating waves at a metallic interface or as localized excitations of a nanoparticle or nanostructure. Plasmonic structures can efficiently couple energy from freely propagating electromagnetic waves to localized electromagnetic fields and vice-versa, essentially acting as an optical antenna. As a result, the intensity of the local fields around and inside the nanostructure are strongly enhanced compared to the incident radiation. In this thesis, this ability to manipulate electromagnetic fields on the nanoscale is employed to control a wide range of optical phenomena. These studies are performed using structures based on metallic nanoshells, which consist of a thin Au shell coating a silica nanosphere. To investigate the parameters controlling the plasmonic response of metallic nanoshells, two changes to the nanoshell composition are studied: (1) the Au shell is replaced with Cu which has interband transitions that strongly influence the plasmon resonance, and (2) the silica core is replaced by a semiconducting CU2O core Ill which has a significantly higher dielectric constant and non-trivial absorbance. The focusing of electromagnetic energy into intense local fields by plasmonic nanostructures is then directly investigated by profiling the nanoshell near field using a Raman-based molecular ruler. Next, plasmons supported by Au nanoshells are used to control the fluorescence of near-infrared fluorophores placed at controlled distances from the nanoshell surface. In this context, the analogy of an optical antenna is very relevant: the enhanced field at the surface of the nanoshell increases the absorption of light by the fluorophore, or equivalently couples propagating electromagnetic waves into a localized receiver, while the large scattering cross section enhances the coupling of energy from a localized source, the fluorophore, to far-field radiation. Excellent agreement with models based on Mie theory is achieved for both Raman and fluorescence. Experimentally measured enhancements of the radiative decay rate for fluorophores on Au nanoshells and Au nanorods are also consistent with this model. Plasmonic nano structures can also control the flow of light into larger structures. This is observed by measuring the nanoparticle-induced enhancement and suppression of photocurrent in a silicon photodiode is at the single particle level for silica nanospheres, Au nanospheres, and two types of Au nanoshell. Finally, the simultaneous physical manipulation of an individual plasmonic nanostructure on the few-nanometer scale using light and detection of the local electromagnetic field during this ongoing process with the same incident beam is performed. For this experiment, a Au nanoshell is separated from a metallic surface by a few-nanometer thick polymer layer to form a nanoscale junction, or nanogap. Illuminating this structure with ultrashort optical pulses, exciting the plasmon resonance, results in a continuous, monitorable collapse of the nanogap. An easily detectable four- IV wave mixing (FWM) signal is simultaneously generated by this illumination of the nanogap, providing a continuous, highly sensitive optical monitor of the nano gap spacing while it is being optically reduced. The dramatic increase in this signal upon contact provides a clear, unambiguous signal of the gap closing. Acknowledgments First and foremost, I have to thank my parents, Michael and Carolyn Grady, who have served as a constant inspiration to open my mind and think about the world around me. Their example has instilled in me the work ethic that I should never aim for "good enough," rather I should push myself to do the best I can, without which I never would have made it to graduate school. My dad's unwavering willingness to answer questions, help me with chemistry sets and electronics kits, bring me along to help set up undergraduate labs and explain what they were about when I was growing up is what inspired me to follow in his footsteps and study physics. My adviser and mentor, Naomi Halas, and Peter Nordlander have both been instrumental in helping develop the ideas in this thesis. Finally, I'd like to thank all the members of the Halas group, whose ideas, criticisms, and support has helped refine the ideas in this thesis. Finally, I have to thank quite a few people who helped me make it through the traumatic events in my personal life over the last year including my family, especially my parents, siblings, aunt Marie and uncle Tom who all came down to Houston; my advisor Naomi Halas who has been unendingly supportive and understanding; and all the members of the Halas group. Table of Contents Chapter 1. Introduction 1 Chapter 2. Electromagnetic properties of nanoparticles 4 2.1 Plasmon hybridization 4 2.2 Mie theory 8 2.3 Surface-enhanced Raman scattering 9 2.4 Fluorescence Enhancement by Plasmonic Nanoantennas 11 Chapter 3. Cu nanoshells: effects of interband transitions on the nanoparticle plasmon resonance 13 Chapter 4. Metallic nanoshells with semiconducting CU2O cores 26 4.1 Results and Discussion 30 4.2 Conclusions 56 Chapter 5. ProfilinRulers g the Near Field of a Nanoshell with Raman-Based Molecular 57 Chapter 6. Nanoscale Control of Near-Infrared Fluorescence Enhancement Using Au Nanoshells 69 6.1 Fabrication of Nanoshell-ICG Conjugates 73 6.1.1 NS@Si02 fabrication: 73 6.1.2 ICG Binding to NS@Si02 Nanoparticles: 74 6.2 Characterization of Nanoshell-ICG Conjugates 75 6.3 Experimental Fluorescence Enhancement 78 6.4 Calculated Fluorescence Enhancement 80 6.5 Calculated Quantum Yield and Lifetime 85 6.6 Conclusions 86 Chapter 7. FluorescencNanorods e Enhancement by Au nanostructures: Nanoshells and 88 VII 7.1 Introduction 88 7.2 Fabrication of Nanoparticle-Fluorophore Conjugates 92 7.2.1 Nanoparticle Fabrication 92 7.2.2 Protein and Fluorophore Conjugation 93 7.2.3 Protein-Fluorophore Complex Binding to Nanoparticles 94 7.2.4 Calculation of surface area available for HSA-IR800 to bind to nanoparticles 95 7.2.5 Calculation of no. of HSA-IR800 molecules bound to the nanoparticles 96 7.3 Characterization of Nanoparticle-Fluorophore Conjugates 97 7.4 Experimental Fluorescence Enhancement 101 7.5 Calculated and Experimental Scattering Efficiency of Nano structures 102 7.6 Frequency Domain Lifetime Decay 105 7.7 Experimental Quantification of Quantum Yield 109 7.8 Conclusions 113 Chapter 8. Nanoparticle-induced Enhancement and Suppression of Photocurrent in a Silicon Photodiode 114 8.1 Structure 116 8.2 Measurement 119 8.3 Results 121 8.4 Conclusions 130 Chapter 9. Optically-Driven Collapse of a Plasmonic Nanogap Self-monitored by Optical Frequency Mixing 132 9.1 Apparatus 135 9.2 Sample Fabrication 139 9.3 Results 140 9.4 Conclusions 149 VIII Chapter 10. Bibliography 150 List of Figures Figure 2-1 Incompressible fluid model for oscillating plasmons in a solid metallic sphere. The metal (green) is composed of a fixed ion background (blue) and an oscillating electron fluid (yellow) 4 Figure 2-2 (A) Schematic diagram of a nanoshell. (B) Hybridization diagram for a Au shell - SiC>2 core nanoshell 5 Figure 2-3 Horizontal lines: dipole plasmon resonance for a Au sphere immersed in air (em = 1.00) or H2O (em = 1.77). Blue curve: dipole plasmon resonance for a spherical cavity in Au (cos) as a function of the dielectric constant (ec) of the material inside the cavity. Points corresponding to a silica core (ec = 2.04, •), a CU2O core (ec ^ 7.1 to 9.1, —), and an iron oxide core (ec ~ 12, •) are indicated in black along the cavity plasmon line 6 Figure 2-4 Energy level diagram for Raman scattering 10 Figure 3-1 Theoretically calculated far field extinction of a solid nanosphere (black solid curves) and nanoshell (blue solid curves) made of (A) Ag, (B) Au, and (C) Cu. The nanospheres are 20 nm in radius and the nanoshells are 63 nm in core (silica) radius and 10 nm in shell thickness. The refractive index of ethanol (n = 1.36) is used as the refractive index of the medium surrounding the particles. Dielectric function (red curves) of (A) Ag, (B) Au, and (C) Cu are also shown, e' and e " are the real and imaginary parts of the dielectric constants, respectively. The green shaded areas indicate the wavelength regions where interband transitions of the metals occur.
Details
-
File Typepdf
-
Upload Time-
-
Content LanguagesEnglish
-
Upload UserAnonymous/Not logged-in
-
File Pages186 Page
-
File Size-