The Function of Bursts of Spikes During Visual Fixation in the Awake Primate Lateral Geniculate Nucleus and Primary Visual Cortex
Total Page:16
File Type:pdf, Size:1020Kb
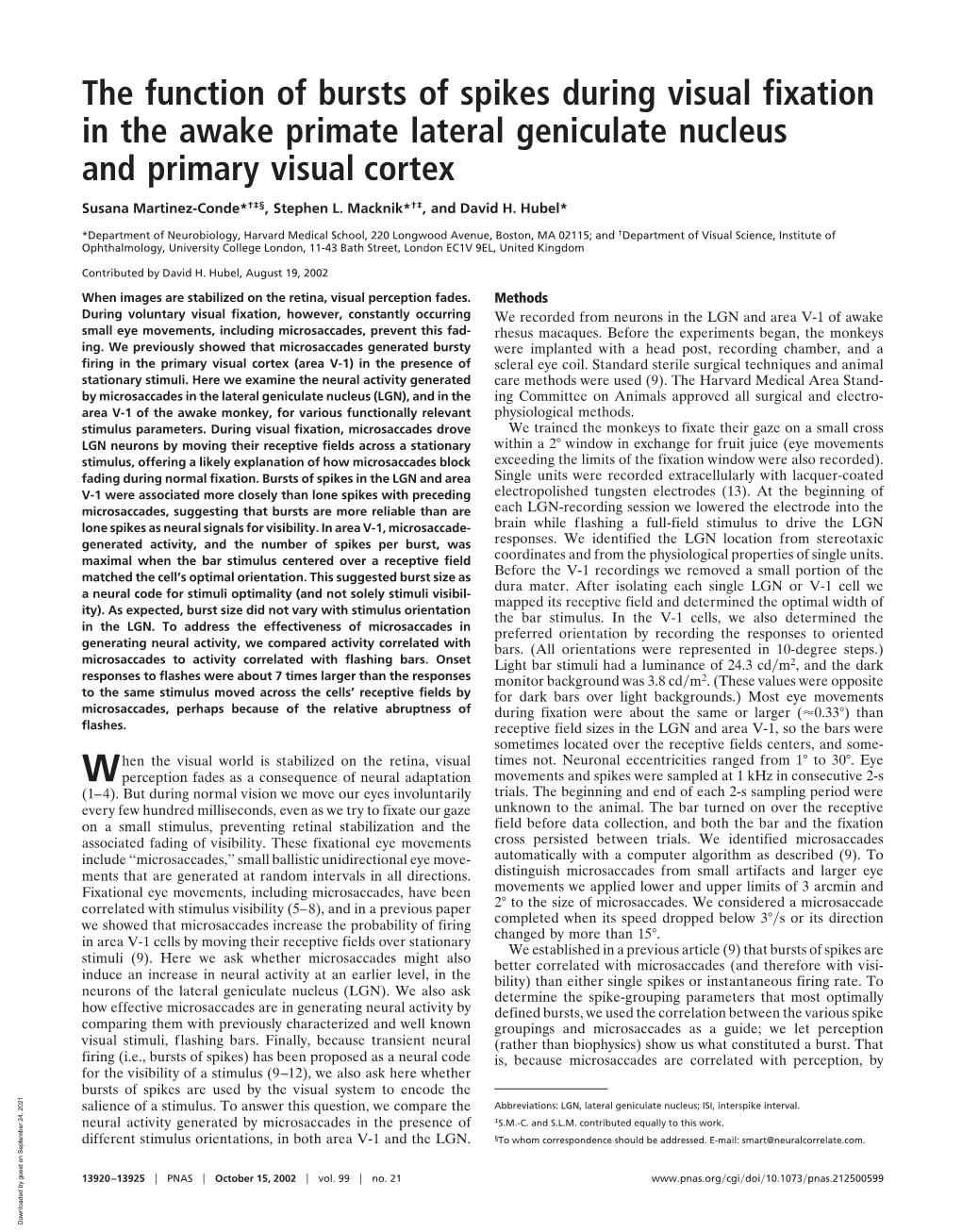
Load more
Recommended publications
-
Subliminal Afterimages Via Ocular Delayed Luminescence: Transsaccade Stability of the Visual Perception and Color Illusion
ACTIVITAS NERVOSA SUPERIOR Activitas Nervosa Superior 2012, 54, No. 1-2 REVIEW ARTICLE SUBLIMINAL AFTERIMAGES VIA OCULAR DELAYED LUMINESCENCE: TRANSSACCADE STABILITY OF THE VISUAL PERCEPTION AND COLOR ILLUSION István Bókkon1,2 & Ram L.P. Vimal2 1Doctoral School of Pharmaceutical and Pharmacological Sciences, Semmelweis University, Budapest, Hungary 2Vision Research Institute, Lowell, MA, USA Abstract Here, we suggest the existence and possible roles of evanescent nonconscious afterimages in visual saccades and color illusions during normal vision. These suggested functions of subliminal afterimages are based on our previous papers (i) (Bókkon, Vimal et al. 2011, J. Photochem. Photobiol. B) related to visible light induced ocular delayed bioluminescence as a possible origin of negative afterimage and (ii) Wang, Bókkon et al. (Brain Res. 2011)’s experiments that proved the existence of spontaneous and visible light induced delayed ultraweak photon emission from in vitro freshly isolated rat’s whole eye, lens, vitreous humor and retina. We also argue about the existence of rich detailed, subliminal visual short-term memory across saccades in early retinotopic areas. We conclude that if we want to understand the complex visual processes, mere electrical processes are hardly enough for explanations; for that we have to consider the natural photobiophysical processes as elaborated in this article. Key words: Saccades Nonconscious afterimages Ocular delayed bioluminescence Color illusion 1. INTRODUCTION Previously, we presented a common photobiophysical basis for various visual related phenomena such as discrete retinal noise, retinal phosphenes, as well as negative afterimages. These new concepts have been supported by experiments (Wang, Bókkon et al., 2011). They performed the first experimental proof of spontaneous ultraweak biophoton emission and visible light induced delayed ultraweak photon emission from in vitro freshly isolated rat’s whole eye, lens, vitreous humor, and retina. -
Dependence of the Stimulus-Driven Microsaccade Rate Signature on 2 Visual Stimulus Polarity 3 4 Tatiana Malevich1,2,3*, Antimo Buonocore1,2, & Ziad M
bioRxiv preprint doi: https://doi.org/10.1101/2020.05.23.112417; this version posted May 25, 2020. The copyright holder for this preprint (which was not certified by peer review) is the author/funder, who has granted bioRxiv a license to display the preprint in perpetuity. It is made available under aCC-BY 4.0 International license. 1 Dependence of the stimulus-driven microsaccade rate signature on 2 visual stimulus polarity 3 4 Tatiana Malevich1,2,3*, Antimo Buonocore1,2, & Ziad M. Hafed1,2 5 6 1Werner Reichardt Centre for Integrative Neuroscience, Tuebingen University, Tuebingen, Germany 7 72076 8 2Hertie Institute for Clinical Brain Research, Tuebingen University, Tuebingen, Germany 72076 9 3Graduate School of Neural and Behavioural Sciences, International Max-Planck Research School, 10 Tuebingen University, Tuebingen, Germany 72076 11 12 * Correspondence to: 13 [email protected] 14 15 Abbreviated title: 16 Black versus white stimulus onset effects on microsaccades 17 18 Corresponding author address: 19 Tatiana Malevich 20 Werner Reichardt Centre for Integrative Neuroscience 21 and 22 Hertie Institute for Clinical Brain Research 23 Otfried-Mueller Str. 25 24 Tuebingen, 72076 25 Germany 26 Phone: +49 7071 29 88821 27 28 29 1 bioRxiv preprint doi: https://doi.org/10.1101/2020.05.23.112417; this version posted May 25, 2020. The copyright holder for this preprint (which was not certified by peer review) is the author/funder, who has granted bioRxiv a license to display the preprint in perpetuity. It is made available under aCC-BY 4.0 International license. 30 Abstract 31 Microsaccades have a steady rate of occurrence during maintained gaze fixation, 32 which gets transiently modulated by abrupt sensory stimuli. -
Steinbach (1972) Release of Pursuit Eye Movements Using After-Images
VisionRcs. Vol. 12, pp. 1307-1311.Pergamon Prss 1972.Printed in Great Britain. LElTER TO THE EDITORS RELEASE OF PURSUIT EYE MOVEMENTS USING AFTER-IMAGES’ (Receioed 8 October 1971) IT IS frequently asserted that a moving visual target is necessary in order for the eyes to make slow, conjugate pursuit movements (e.g. ROBINSON,1968). There are, however, viola- tions to this fiat (see, e.g. RICHARDSand STEINBACH,1972; YOUNG, 1971). Some subjects can smoothly track their own moving hand in complete darkness (STEINBACH,1969; VON NMRDEN and MACKENSEN,1962), indicating that an internal proprioceptive motion signal may be sufficient for pursuit. Another far more commonly observed exception concerns images rendered stable with respect to the retina. Several investigators (HEDLUNand WHITE, 1959; RIGGS and TULUNAY,1959; TEN DOESSCHATE,1954) have shown that optically stabi- lized images may produce smooth oscillations of the eyes. After-images provide another example where smooth movements can be made without any moving visual target (MACK and BACHANT,1969). Why should stable images on the retina allow the release of pursuit eye movements? This poses a conceptual problem because, when done over a homogeneous or dark back- ground, no retinal signal for motion can be present. What then is the stimulus for pursuit with a stabilized image? How can the pursuit movement begin? One testable hypothesis concerns the “outflow” or “corollary discharge” model postu- lated to account for the stability of the visual world during eye movements (see, e.g. TEUBER, 1960). It is assumed that for voluntary eye movements, a corollary discharge is issued along with the efferent signal sent to the eye muscles so that the visual reafference resulting from the movement can be cancelled. -
Bayesian Microsaccade Detection
Journal of Vision (2017) 17(1):13, 1–23 1 Bayesian microsaccade detection * Center for Neural Science, New York University, Andra Mihali New York, NY, USA $ * Center for Neural Science, New York University, Bas van Opheusden New York, NY, USA $ Center for Neural Science, New York University, New York, NY, USA Department of Psychology, New York University, Wei Ji Ma New York, NY, USA $ Microsaccades are high-velocity fixational eye Introduction movements, with special roles in perception and cognition. The default microsaccade detection method is to determine when the smoothed eye velocity Even when people attempt to fixate, their eyes are exceeds a threshold. We have developed a new always in motion. Fixational eye movements are often method, Bayesian microsaccade detection (BMD), categorized as drift, tremor, and microsaccades (Ciuf- which performs inference based on a simple freda & Tannen, 1995). During most of the fixation statistical model of eye positions. In this model, a time, the eye is in a drift state, which is low-amplitude, hidden state variable changes between drift and low-velocity motion, sometimes modeled as a random microsaccade states at random times. The eye walk (Cornsweet, 1956; Ditchburn & Ginsborg, 1953; position is a biased random walk with different Ratliff & Riggs, 1950). A few times a second, the eye velocity distributions for each state. BMD generates makes a microsaccade, which is a high-velocity samples from the posterior probability distribution movement along a relatively straight trajectory (Corn- overtheeyestatetimeseriesgiventheeyeposition sweet, 1956; Engbert & Kliegl, 2003; Engbert, Mer- timeseries.Appliedtosimulateddata,BMDrecovers genthaler, Sinn, & Pikovsky, 2011; Rolfs, 2009). the ‘‘true’’ microsaccades with fewer errors than Microsaccades have been implicated in several alternative algorithms, especially at high noise. -
Visual Vs. Spatial Contributions to Microsaccades and Visual-Spatial Working Memory
Journal of Eye Movement Research (2014) 7(2):2, 1-14 Visual vs. Spatial Contributions to Microsaccades and Visual-Spatial Working Memory Joshua T. Gaunt Bruce Bridgeman Department of Psychology University of California, Santa Cruz Microsaccade rates and directions were monitored while observers performed a visual work- ing memory task at varying retinal eccentricities. We show that microsaccades generate no in- terference in a working memory task, indicating that spatial working memory is at least par- tially insulated from oculomotor activity. Intervening tasks during the memory interval af- fected microsaccade patterns; microsaccade frequency was consistently higher during concur- rent spatial tapping (no visual component) than during exposure to dynamic visual noise (no task). Average microsaccade rate peaked after appearance of a fixation cross at the start of a trial, and dipped at cue onset and offset, consistent with previous results. Direction of stimuli in choice tasks did not influence microsaccade direction, however. Keywords: Microsaccades, attention, working memory, dynamic visual noise, concurrent spatial tapping (Tresch et al., 1993) isolated the distinction between There is extensive evidence for the existence of two visual and spatial STM from a number of alternative visual streams, where ventral projections from V1 explanations. Their spatial task measured memory for serve cognitive aspects of perception while parietal a dot location. The visual memory task in contrast had cortex serves visually guided behavior, or "what" and participants select a Chinese ideogram that had been "how" systems respectively (Bridgeman, Lewis, Heit shown previously. Both tasks had 10 sec delays and & Nagle, 1975; Bridgeman, Kirch, & Sperling, 1981; seven distractor locations or ideograms at test. -
Microsaccade Production During Saccade Cancelation in a Stop-Signal Task ⇑ David C
Vision Research 118 (2016) 5–16 Contents lists available at ScienceDirect Vision Research journal homepage: www.elsevier.com/locate/visres Microsaccade production during saccade cancelation in a stop-signal task ⇑ David C. Godlove, Jeffrey D. Schall Department of Psychology, Vanderbilt Vision Research Center, Center for Integrative & Cognitive Neuroscience, Vanderbilt Brain Institute, Vanderbilt University, Nashville, TN 37240, USA article info abstract Article history: We obtained behavioral data to evaluate two alternative hypotheses about the neural mechanisms of Received 10 July 2014 gaze control. The ‘‘fixation’’ hypothesis states that neurons in rostral superior colliculus (SC) enforce fix- Received in revised form 13 October 2014 ation of gaze. The ‘‘microsaccade’’ hypothesis states that neurons in rostral SC encode microsaccades Available online 6 November 2014 rather than fixation per se. Previously reported neuronal activity in monkey SC during the saccade stop-signal task leads to specific, dissociable behavioral predictions of these two hypotheses. When sub- Keywords: jects are required to cancel partially-prepared saccades, imbalanced activity spreads across rostral and Rostral superior colliculus caudal SC with a reliable temporal profile. The microsaccade hypothesis predicts that this imbalance will Frontal eye field lead to elevated microsaccade production biased toward the target location, while the fixation hypothesis Fixation neurons Premotor theory of attention predicts reduced microsaccade production. We tested these predictions by analyzing the microsaccades Response inhibition produced by 4 monkeys while they voluntarily canceled partially prepared eye movements in response to explicit stop signals. Consistent with the fixation hypothesis and contradicting the microsaccade hypoth- esis, we found that each subject produced significantly fewer microsaccades when normal saccades were successfully canceled. -
Limitations on Fixation Stability and Acuity?
Vision Research 114 (2015) 87–99 Contents lists available at ScienceDirect Vision Research journal homepage: www.elsevier.com/locate/visres Characteristics of fixational eye movements in amblyopia: Limitations on fixation stability and acuity? ⇑ Susana T.L. Chung , Girish Kumar, Roger W. Li, Dennis M. Levi School of Optometry, University of California Berkeley, Berkeley, CA 94720-2020, United States article info abstract Article history: Persons with amblyopia, especially those with strabismus, are known to exhibit abnormal fixational eye Received 16 September 2014 movements. In this paper, we compared six characteristics of fixational eye movements among normal Received in revised form 22 January 2015 control eyes (n = 16), the non-amblyopic fellow eyes and the amblyopic eyes of anisometropic (n = 14) Available online 7 February 2015 and strabismic amblyopes (n = 14). These characteristics include the frequency, magnitude of landing errors, amplitude and speed of microsaccades, and the amplitude and speed of slow drifts. Fixational Keywords: eye movements were recorded using retinal imaging while observers monocularly fixated a 1° cross. Amblyopia Eye position data were recovered using a cross-correlation procedure. We found that in general, the char- Fixational eye movements acteristics of fixational eye movements are not significantly different between the fellow eyes of amblyo- Fixation stability Visual acuity pes and controls, and that the strabismic amblyopic eyes are always different from the other groups. Microsaccades Next, we determined the primary factors that limit fixation stability and visual acuity in amblyopic eyes Slow drifts by examining the relative importance of the different oculomotor characteristics, adding acuity (for fixation stability) or fixation stability (for acuity), and the type of amblyopia, as predictive factors in a multiple linear regression model. -
Investigating the Relationship Between Microsaccades
INVESTIGATING THE RELATIONSHIP BETWEEN MICROSACCADES AND OSCILLATIONS IN THE HUMAN VISUAL CORTEX by Kacper Wieczorek Supervisors: Krish D. Singh & Petroc Sumner A thesis submitted to Cardiff University for the degree of Doctor of Philosophy. © Copyright by Kacper Wieczorek, September 2015 Summary Neural oscillations play important roles in vision and attention. Most studies of oscillations use visual fixation to control the visual input. Small eye movements, called microsaccades, occur involuntarily ~ 1-2 times per second during fixation and they are also thought to play important roles in vision and attention. The aim of the work described in this thesis was to explore the relationship between microsaccades and oscillations in the human visual cortex. In Chapter 2, I describe how remote video eye tracking can be used to detect and characterize microsaccades during MEG recordings. Tracking based on the pupil position only, without corneal reflection, and with the participant’s head immobilized in the MEG dewar, resulted in high precision gaze tracking and enabled the following investigations. In Chapter 3, I investigated the relationship between induced visual gamma oscillations and microsaccades in a simple visual stimulation paradigm. I did not find evidence for the relationship. This finding supports the view that sustained gamma oscillations reflect local processing in cortical columns. In addition, early transient gamma response had a reduced amplitude on trials with microsaccades, however the exact nature of this effect will have to be determined in future studies. In Chapter 4, I investigated the relationship between alpha oscillations and microsaccades in covert spatial attention. I did not find evidence for a relationship between hemispheric lateralization of the alpha amplitude and the directional bias of microsaccades. -
Microsaccade Control Signals in the Cerebellum
The Journal of Neuroscience, February 25, 2015 • 35(8):3403–3411 • 3403 Behavioral/Cognitive Microsaccade Control Signals in the Cerebellum X Daniel Arnstein,1,2 Marc Junker,1,2 Aleksandra Smilgin,1,2 Peter W. Dicke,1 and Peter Thier1 1Department of Cognitive Neurology, Hertie Institute for Clinical Brain Research, Tu¨bingen 72076, Germany; and 2Graduate School of Neural and Behavioural Sciences, University of Tu¨bingen, Tu¨bingen 72074, Germany Microsaccades, the small saccades made when we try to keep the eyes still, were once believed to be inconsequential for vision, but recent studies suggest that they can precisely relocate gaze to tiny visual targets. Because the cerebellum is necessary for motor precision, we investigated whether microsaccades may exploit this neural machinery in monkeys. Almost all vermal Purkinje cells, which provide the eye-related output of the cerebellar cortex, were found to increase or decrease their simple spike firing rate during microsaccades. At both the single-cell and population level, microsaccade-related activity was highly similar to macrosaccade-related activity and we observed a continuous representation of saccade amplitude that spanned both the macrosaccade and microsaccade domains. Our results suggest that the cerebellum’s role in fine-tuning eye movements extends even to the oculomotor system’s smallest saccades and add to a growing list of observations that call into question the classical categorical distinction between microsaccades and macrosaccades. Key words: cerebellum; fixational eye movements; microsaccades; oculomotor; Purkinje cells Introduction al., 1973), that they are not necessary for maintaining accurate In primates, high-acuity vision is limited to the fovea. -
When Do Microsaccades Follow Spatial Attention?
Attention, Perception, & Psychophysics 2010, 72 (3), 683-694 doi:10.3758/APP.72.3.683 When do microsaccades follow spatial attention? JOCHEN LAUBROCK AND REINHOLD KLIEGL University of Potsdam, Potsdam, Germany MARTIN ROLFS University of Potsdam, Potsdam, Germany and CNRS and Université Paris Descartes, Paris, France AND RALF ENGBERT University of Potsdam, Potsdam, Germany Following up on an exchange about the relation between microsaccades and spatial attention (Horowitz, Fenc- sik, Fine, Yurgenson, & Wolfe, 2007; Horowitz, Fine, Fencsik, Yurgenson, & Wolfe, 2007; Laubrock, Engbert, Rolfs, & Kliegl, 2007), we examine the effects of selection criteria and response modality. We show that for Posner cuing with saccadic responses, microsaccades go with attention in at least 75% of cases (almost 90% if probability matching is assumed) when they are first (or only) microsaccades in the cue–target interval and when they occur between 200 and 400 msec after the cue. The relation between spatial attention and the direction of microsaccades drops to chance level for unselected microsaccades collected during manual-response conditions. Analyses of data from four cross-modal cuing experiments demonstrate an above-chance, intermediate link for visual cues, but no systematic relation for auditory cues. Thus, the link between spatial attention and direction of microsaccades depends on the experimental condition and time of occurrence, but it can be very strong. Eye movements and visual attention are intimately fixations. Since the absence of saccades during covert at- related. The major function of saccadic eye movements tention shifts does not imply the absence of fixational eye is to move objects of interest into the fovea, the retinal movements, microsaccades—small saccade-like move- region of highest acuity, for close inspection during the ments with amplitudes 1º that occur during attempted following fixation (Findlay & Gilchrist, 2003). -
The Multifunctional Lateral Geniculate Nucleus
Rev. Neurosci. 2016; 27(2): 135–157 Open Access Theodore G. Weyand* The multifunctional lateral geniculate nucleus DOI 10.1515/revneuro-2015-0018 the LGN that is often promoted as the prototype dorsal tha- Received May 11, 2015; accepted September 1, 2015; previously lamic nucleus (e.g. Sherman and Guillery, 2004). The LGN published online October 17, 2015 is that thalamic structure that receives from the retina and projects to visual cortex. Because the LGN neurons project- Abstract: Providing the critical link between the retina and ing to cortex are also retinal-recipient, because the retina visual cortex, the well-studied lateral geniculate nucleus drives these neurons, and because the receptive field struc- (LGN) has stood out as a structure in search of a function ture of these neurons appears nearly the same as the retinal exceeding the mundane ‘relay’. For many mammals, it is ganglion cells driving them (concentric), the cells and the structurally impressive: Exquisite lamination, sophisti- structure are tagged ‘relay’, implying that nothing happens. cated microcircuits, and blending of multiple inputs sug- Given the sophisticated anatomy and exquisite microcir- gest some fundamental transform. This impression is cuits, this is a big disappointment. Sherman and Guillery bolstered by the fact that numerically, the retina accounts (1996) lament that the thalamus, and the LGN in particu- for a small fraction of its input. Despite such promise, the lar, suffers from ‘bad press’. Possibly, but, if the LGN truly extent to which an LGN neuron separates itself from its has an undeserved image problem, the solution seems not retinal brethren has proven difficult to appreciate. -
Do Eye Movements Enhance Visual Memory Retrieval?
Vision Research 176 (2020) 80–90 Contents lists available at ScienceDirect Vision Research journal homepage: www.elsevier.com/locate/visres Do eye movements enhance visual memory retrieval? T ⁎ Hikari Kinjoa,b, , Jolande Fookenb,c, Miriam Speringb,c,d a Faculty of Psychology, Meiji Gakuin University, Tokyo, Japan b Dept Ophthalmology & Visual Sciences, University of British Columbia, Vancouver, BC, Canada c Institute for Computing, Information and Cognitive Systems, University of British Columbia, Vancouver, BC, Canada d Center for Brain Health, University of British Columbia, Vancouver, BC, Canada ARTICLE INFO ABSTRACT Keywords: When remembering an object at a given location, participants tend to return their gaze to that location even after Eye movements the object has disappeared, known as Looking-at-Nothing (LAN). However, it is unclear whether LAN is asso- Looking at nothing ciated with better memory performance. Previous studies reporting beneficial effects of LAN have often not Memory retrieval systematically manipulated or assessed eye movements. We asked 20 participants to remember the location and Saccades identity of eight objects arranged in a circle, shown for 5 s. Participants were prompted to judge whether a Microsaccades location statement (e.g., “Star Right”) was correct or incorrect, or referred to a previously unseen object. During memory retrieval, participants either fixated in the screen center or were free to move their eyes. Results reveal no difference in memory accuracy and response time between free-viewing and fixation while a LAN effect was found for saccades during free viewing, but not for microsaccades during fixation. Memory performance was better in those free-viewing trials in which participants made a saccade to the critical location, and scaled with saccade accuracy.