Lipid-Mediated Protein Functionalization of Electrospun Polycaprolactone Fibers
Total Page:16
File Type:pdf, Size:1020Kb
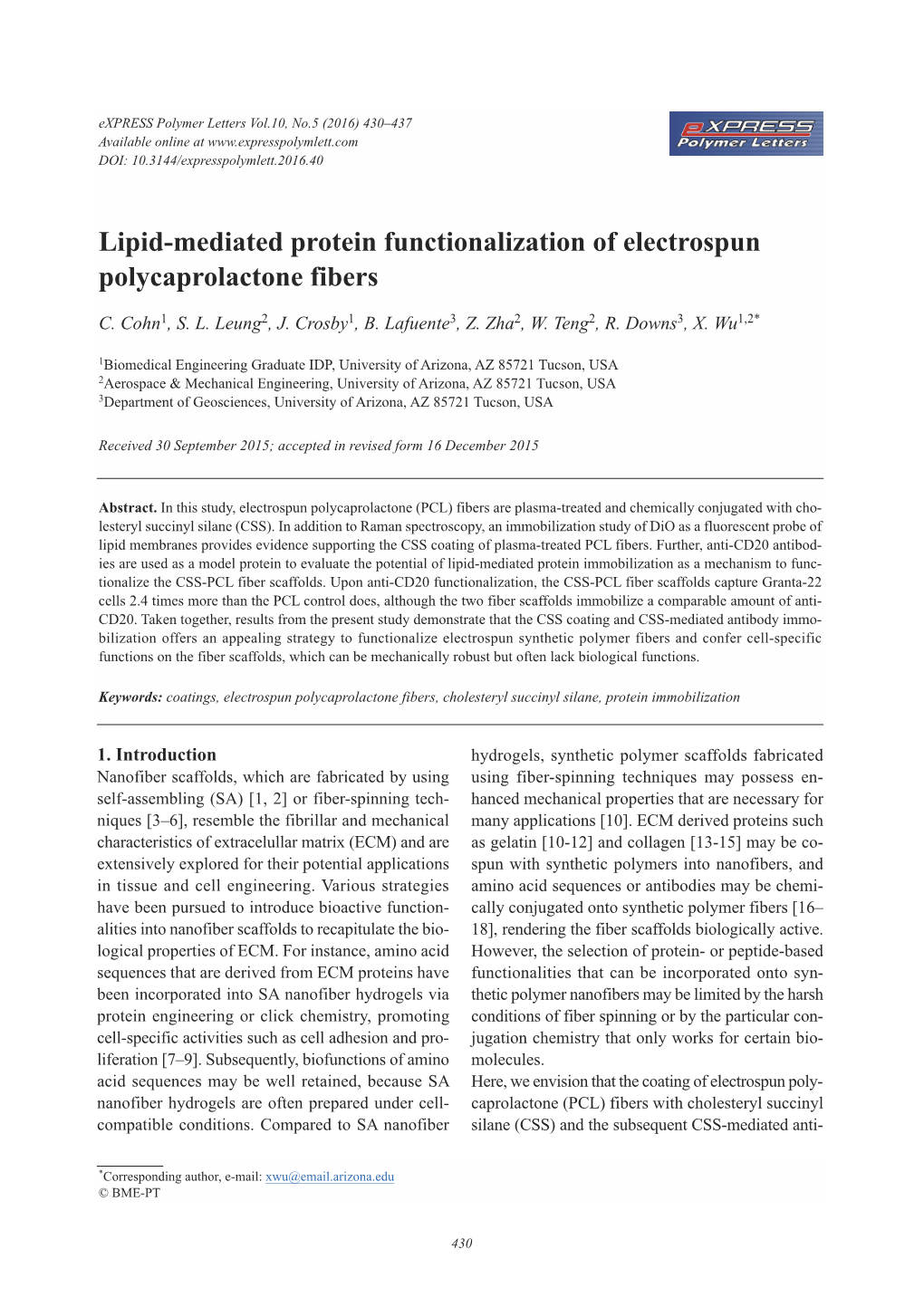
Load more
Recommended publications
-
Chitin Nanofibers, Networks and Composites
Chitin nanofibers, networks and composites - Preparation, structure and mechanical properties Ngesa Ezekiel Mushi KTH, Royal Institute of Technology School of Chemical Science and Engineering Department of Fibre and Polymer Technology Division of Biocomposites Doctoral Thesis Stockholm 2014 Supervisor Prof Lars A Berglund Copyright © Ngesa Ezekiel Mushi, Stockholm 2014 All rights reserved The following papers are reprinted with permission: Paper I © 2014 Wiley Periodicals, Inc. Paper II © 2014 Elsevier Ltd. Paper III © 2014 Mushi, Utsel and Berglund - Frontiers Paper IV © 2014 Manuscript Paper V © 2014 Manuscript TRITA-CHE Report 2014:43 ISSN 1654-1081 ISBN 978-91-7595-312-0 Tryck: US-AB, Stockholm 2014 AKADEMISK AVHANDLING Som med tillstånd av Kungliga Tekniska Högskolan framläggs till offentlig granskning för avläggande av teknisk doktorsexamen i fiber och polymerteknologi fredagen den 28 november 2014, kl 13:00 i sal Kollegiesalen, Brinellvägen 8, KTH campus, Stockholm. Fakultetsopponent: Prof Shinsuke Ifuku från Tottori University in Japan. Avhandlingen försvaras på engelska. Wakunde baba na mamaakwa walemiinutwa na nkaakwa nkunde na wana wakwa wesha Reuben na Ulla ABSTRACT Chitin is an important reinforcing component in load-bearing structures in many organisms such as insects and crustaceans (i.e. shrimps, lobsters, crabs etc.). It is of increasing interest for use in packaging materials as well as in biomedical applications. Furthermore, biological materials may inspire the development of new man-made material concepts. Chitin molecules are crystallized in extended chain conformations to form nanoscale fibrils of about 3 nm in diameter. In the present study, novel materials have been developed based on a new type of chitin nanofibers prepared from the lobster exoskeleton. -
Nanofiber Solutions Brochure
Nanofiber Solutions || 1 Nanofiber Solutions || 2 Table of Contents Introduction .............................................................................................................. 3 Research Areas ........................................................................................................ 4 Cancer Research .......................................................................................... 4 Stem Cell Research ..................................................................................... 4 Cell Migration Analysis ........................................................................ 5 In Vitro Disease Models ............................................................................... 8 Tissue Engineering Scaffolds ..................................................................... 8 Schwann Cell Alignment ..................................................................... 9 High Throughput Drug Discovery .............................................................. 11 Brain Cancer Drug Sensitivity ........................................................... 12 Successful Manufacturing of Artificial Trachea .................................................. 17 Nanofiber Comparisons to Native Tissue ............................................................. 18 Products .................................................................................................................... 19 Cell Seeding Protocol ............................................................................................. -
Preparations and Characterization of Chitin Nanofibers and Related Materials Ajoy Kumar Dutta
Preparations and Characterization of Chitin Nanofibers and Related Materials July, 2014 Ajoy Kumar Dutta Department of Chemistry and Biotechnology Graduate School of Engineering Tottori University CONTENTS General Introduction 1 Chapter1. Preparation of α-Chitin Nanofibers by High Pressure Water Jet System: Impact of Number of Passes on Nanofibrillation 6 1.1 Introduction 1.2 Experimental 1.3 Results and Discussion 1.4 Conclusion Chapter 2. Simple Preparation of β-Chitin Nanofibers from Squid Pen 26 2.1 Introduction 2.2 Experimental 2.3 Results and Discussion 2.4 Conclusion Chapter 3. Novel Preparation of Chitin Nanocrystals by H2SO4 and H3PO4 Hydrolysis 40 3.1 Introduction 3.2 Experimental 3.3 Results and Discussion 3.4 Conclusion -I- Chapter 4. Simple Preparation of Chitosan Nanofibers by High Pressure Water Jet System 55 4.1 Introduction 4.2 Experimental 4.3 Results and Discussion 4.4 Conclusion Chapter 5. Facile Preparation of Surface N-halamine Chitin Nanofiber to Endow Antimicrobial Activities 70 5.1 Introduction 5.2 Experimental 5.3 Results and Discussion 5.4 Conclusion General Summary 88 References 92 List of Publications 106 Acknowledgements 107 -II- List of Figures Figure 1. Chemical structure of (a) cellulose, (b) chitin, and (c) chitosan. 2 Figure 2. FE-SEM micrograph of α-chitin powder. 8 Figure 3. FE-SEM micrographs of α-chitin fibers after several passes treatments by HPWJ system. The scale bar length is 400 nm. 14 Figure 4. FT-IR spectrum of α-chitin nanofibers at several passes. 15 Figure 5. Average widths of α-chitin nanofibers as a function of number of passes. -
Nanofibers Based on Concentrated Collagen Hydrolysate Loaded with Essential Oils
https://www.scientificarchives.com/journal/journal-of-nanotechnology-and-nanomaterials Journal of Nanotechnology and Nanomaterials Research Article Nanofibers Based on Concentrated Collagen Hydrolysate Loaded with Essential Oils M. D. Berechet1, C. Gaidau1, A. Miletic2, B. Pilic2, D. Simion1, M. Râpă3, M. Stanca1, R. Constantinescu1 1Leather and Footwear Research Institute Division, National Research and Development Institute for Textiles and Leather, Bucharest, Romania 2Faculty of Technology, University of Novi Sad, Novi Sad, Serbia 3Center for Research and Eco-Metallurgical Expertise, Politehnica University of Bucharest, Bucharest, Romania *Correspondence should be addressed to M. D. Berechet; [email protected] Received date: November 24, 2020, Accepted date: December 22, 2020 Copyright: © 2021 Berechet MD, et al. This is an open-access article distributed under the terms of the Creative Commons Attribution License, which permits unrestricted use, distribution, and reproduction in any medium, provided the original author and source are credited. Abstract Collagen is a biopolymer with regenerative and tissue reconstruction properties used in various treatments in medicine, but few research studies were dedicated to the electrospinning of collagen derivatives loaded with essential oils as efficient antimicrobial biomaterial. This research aimed to obtain antibacterial nanofibers based on concentrated collagen hydrolysate loaded with cloves and cinnamon essential oils as natural alternatives to synthesis products. The essential oils were successfully incorporated into collagen using electrospinning process, resulting in nanofibers with diameter from 404.8 nm to 717.6 nm and porous structure. The presence of essential oils in collagen nanofiber mats was confirmed by Attenuated Total Reflectance-Fourier Transform Infrared Spectroscopy (ATR-FTIR), Ultraviolet–visible spectroscopy (UV–VIS) and antibacterial activity assays. -
Polymeric Nanofiber Coating with Tunable Combinatorial Antibiotic
Polymeric nanofiber coating with tunable combinatorial PNAS PLUS antibiotic delivery prevents biofilm-associated infection in vivo Alyssa G. Ashbaugha,1, Xuesong Jiangb,c,d,1, Jesse Zhenge, Andrew S. Tsaie, Woo-Shin Kima, John M. Thompsonf, Robert J. Millera, Jonathan H. Shahbaziana, Yu Wanga, Carly A. Dillena, Alvaro A. Ordonezg,h, Yong S. Changg,h, Sanjay K. Jaing,h, Lynne C. Jonesf, Robert S. Sterlingf, Hai-Quan Maob,c,d,i,2,3, and Lloyd S. Millera,b,f,j,2,3 aDepartment of Dermatology, The Johns Hopkins University School of Medicine, Baltimore, MD 21231; bDepartment of Materials Science and Engineering, Johns Hopkins University, Baltimore, MD 21218; cTranslational Tissue Engineering Center, Johns Hopkins University, Baltimore, MD 21218; dInstitute for NanoBioTechnology, Johns Hopkins University, Baltimore, MD 21218; eDepartment of Biomedical Engineering, Johns Hopkins University, Baltimore, MD 21218; fDepartment of Orthopaedic Surgery, The Johns Hopkins University School of Medicine, Baltimore, MD 21287; gDepartment of Pediatrics, The Johns Hopkins University School of Medicine, Baltimore, MD 21287; hCenter for Infection and Inflammation Imaging Research, The Johns Hopkins University School of Medicine, Baltimore, MD 21287; iWhitaker Biomedical Engineering Institute, Johns Hopkins University, Baltimore, MD 21218; and jDivision of Infectious Diseases, Department of Medicine, The Johns Hopkins University School of Medicine, Baltimore, MD 21287 Edited by Scott J. Hultgren, Washington University School of Medicine, St. Louis, MO, and approved September 20, 2016 (received for review August 23, 2016) Bacterial biofilm formation is a major complication of implantable potential development of antibiotic resistance while on therapy medical devices that results in therapeutically challenging chronic (14–16). infections, especially in cases involving antibiotic-resistant bacteria. -
Chitin and Chitosan Nanofibers: Preparation and Chemical Modifications
Molecules 2014, 19, 18367-18380; doi:10.3390/molecules191118367 OPEN ACCESS molecules ISSN 1420-3049 www.mdpi.com/journal/molecules Review Chitin and Chitosan Nanofibers: Preparation and Chemical Modifications Shinsuke Ifuku Department of Chemistry and Biotechnology, Graduate School of Engineering, Tottori University, 4-101 Koyama-cho Minami, Tottori 680-8550, Japan; E-Mail: [email protected]; Tel.: +81-857-31-5592; Fax: +81-857-31-3190 External Editor: Derek J. McPhee Received: 18 September 2014; in revised form: 15 October 2014 / Accepted: 4 November 2014 / Published: 11 November 2014 Abstract: Chitin nanofibers are prepared from the exoskeletons of crabs and prawns, squid pens and mushrooms by a simple mechanical treatment after a series of purification steps. The nanofibers have fine nanofiber networks with a uniform width of approximately 10 nm. The method used for chitin-nanofiber isolation is also successfully applied to the cell walls of mushrooms. Commercial chitin and chitosan powders are also easily converted into nanofibers by mechanical treatment, since these powders consist of nanofiber aggregates. Grinders and high-pressure waterjet systems are effective for disintegrating chitin into nanofibers. Acidic conditions are the key factor to facilitate mechanical fibrillation. Surface modification is an effective way to change the surface property and to endow nanofiber surface with other properties. Several modifications to the chitin NF surface are achieved, including acetylation, deacetylation, phthaloylation, naphthaloylation, maleylation, chlorination, TEMPO-mediated oxidation, and graft polymerization. Those derivatives and their properties are characterized. Keywords: chitin; chitosan; nanofiber; chemical modification 1. Introduction A nanofiber (NF) is generally defined as a fiber of less than 100 nm diameter and an aspect ratio of more than 100 [1,2]. -
Vertically Aligned Carbon Nanofiber Arrays As a Platform for Gene Delivery and Expression Analysis in Mammalian Cells
University of Tennessee, Knoxville TRACE: Tennessee Research and Creative Exchange Doctoral Dissertations Graduate School 5-2008 Vertically Aligned Carbon Nanofiber Arrays as a Platform for Gene Delivery and Expression Analysis in Mammalian Cells David George James Mann University of Tennessee - Knoxville Follow this and additional works at: https://trace.tennessee.edu/utk_graddiss Part of the Microbiology Commons Recommended Citation Mann, David George James, "Vertically Aligned Carbon Nanofiber Arrays as a Platform for Gene Delivery and Expression Analysis in Mammalian Cells. " PhD diss., University of Tennessee, 2008. https://trace.tennessee.edu/utk_graddiss/362 This Dissertation is brought to you for free and open access by the Graduate School at TRACE: Tennessee Research and Creative Exchange. It has been accepted for inclusion in Doctoral Dissertations by an authorized administrator of TRACE: Tennessee Research and Creative Exchange. For more information, please contact [email protected]. To the Graduate Council: I am submitting herewith a dissertation written by David George James Mann entitled "Vertically Aligned Carbon Nanofiber Arrays as a Platform for Gene Delivery and Expression Analysis in Mammalian Cells." I have examined the final electronic copy of this dissertation for form and content and recommend that it be accepted in partial fulfillment of the equirr ements for the degree of Doctor of Philosophy, with a major in Microbiology. Gary S. Sayler, Major Professor We have read this dissertation and recommend its acceptance: -
New Biocomposite Electrospun Fiber/Alginate Hydrogel for Probiotic Bacteria Immobilization
materials Article New Biocomposite Electrospun Fiber/Alginate Hydrogel for Probiotic Bacteria Immobilization Adam Grzywaczyk 1, Agata Zdarta 1 , Katarzyna Jankowska 1, Andrzej Biadasz 2, Jakub Zdarta 1 , Teofil Jesionowski 1 , Ewa Kaczorek 1 and Wojciech Smułek 1,* 1 Institute of Chemical Technology and Engineering, Faculty of Chemical Technology, Poznan University of Technology, Berdychowo 4, 60-965 Poznan, Poland; [email protected] (A.G.); [email protected] (A.Z.); [email protected] (K.J.); [email protected] (J.Z.); teofi[email protected] (T.J.); [email protected] (E.K.) 2 Institute of Physics, Faculty of Materials Engineering and Technical Physics, Poznan University of Technology, Piotrowo 3, 60-965 Poznan, Poland; [email protected] * Correspondence: [email protected] Abstract: Biotechnological use of probiotic microorganisms involves providing them with appropri- ate conditions for growth, but also protection against environmental changes caused by an exchange of the medium, isolation of metabolites, etc. Therefore, the research on effective immobilization of probiotic microorganisms should be focused in this direction. The present study aimed to evaluate the effectiveness of an innovative hybrid immobilization system based on electrospun nanofibers and alginate hydrogel. The analyses carried out included the study of properties of the initial compo- nents, the evaluation of the degree and durability of cell immobilization in the final material, and their survival under stress conditions. Effective binding of microorganisms to the hydrogel and Citation: Grzywaczyk, A.; Zdarta, nanofibers was confirmed, and the collected results proved that the proposed biocomposite is an A.; Jankowska, K.; Biadasz, A.; efficient method of cell protection. -
Multifunctional Nanoparticles Reinforced Nanofibers by Electrospinning
TH 16 INTERNATIONAL CONFERENCE ON COMPOSITE MATERIALS MULTIFUNCTIONAL NANOPARTICLES REINFORCED NANOFIBERS BY ELECTROSPINNING * Heejae Yang, **Hui Li, **Wei-Heng Shih, ***Yoshihiro Yamashita **Frank Ko : * School of Biomedical Engineering, Science and Health System **Department of Materials Sciences and Engineering Drexel University, Philadelphia, PA ***Department of Materials Science, The University of Shiga Prefecture, Japan Keywords: Electrospinning, Nanofiber, Nanoparticles, Nanocomposites, CdS, Fe3O4 Abstract evident at the size smaller than 100 nm. One of the unique features of nanomaterial is the enormous Nanomaterials in various geometry such as surface area to volume ratio as compared to the bulk quantum-dots, carbon nanotubes and nanoclays material. This can make materials more chemically have been used effectively as coating and fillers for reactive and affect their strength and/or electrical many products to achieve nanoscale effects. In order properties. to amplify the nanoscale effect and provide a means In order to facilitate the translation of to carry the nanoscale effect to macrostructures, the nanoscale effect to macrostructures, creating nanoparticles are incorporated in ultrafine fibers to nanocomposite has been one of the effective form nanocomposite fibrils. This was carried out by methods to utilize the unique properties of nanoscale a co-electrospinning process wherein materials. Among various techniques of fabricating nanocomposite fibrils were spun from a spinning polymer-nanoparticles nanocomposite systems, -
Electrospun Nanofiber Scaffolds: Engineering Soft Tissues
Home Search Collections Journals About Contact us My IOPscience Electrospun nanofiber scaffolds: engineering soft tissues This article has been downloaded from IOPscience. Please scroll down to see the full text article. 2008 Biomed. Mater. 3 034002 (http://iopscience.iop.org/1748-605X/3/3/034002) The Table of Contents and more related content is available Download details: IP Address: 155.37.237.235 The article was downloaded on 12/04/2010 at 15:09 Please note that terms and conditions apply. IOP PUBLISHING BIOMEDICAL MATERIALS Biomed. Mater. 3 (2008) 034002 (15pp) doi:10.1088/1748-6041/3/3/034002 Electrospun nanofiber scaffolds: engineering soft tissues SGKumbar1,RJames2, S P Nukavarapu1 and C T Laurencin1,2,3 1 Department of Orthopaedic Surgery, University of Virginia, VA 22908, USA 2 Department of Biomedical Engineering, University of Virginia, VA 22908, USA 3 Department of Chemical Engineering, University of Virginia, VA 22904, USA E-mail: [email protected] Received 2 August 2007 Accepted for publication 1 October 2007 Published 8 August 2008 Online at stacks.iop.org/BMM/3/034002 Abstract Electrospinning has emerged to be a simple, elegant and scalable technique to fabricate polymeric nanofibers. Pure polymers as well as blends and composites of both natural and synthetics have been successfully electrospun into nanofiber matrices. Physiochemical properties of nanofiber matrices can be controlled by manipulating electrospinning parameters to meet the requirements of a specific application. Such efforts include the fabrication of fiber matrices containing nanofibers, microfibers, combination of nano–microfibers and also different fiber orientation/alignments. Polymeric nanofiber matrices have been extensively investigated for diversified uses such as filtration, barrier fabrics, wipes, personal care, biomedical and pharmaceutical applications. -
ECM Decorated Electrospun Nanofiber for Improving Bone
polymers Article ECM Decorated Electrospun Nanofiber for Improving Bone Tissue Regeneration Yong Fu 1,*, Lili Liu 2, Ruoyu Cheng 2 and Wenguo Cui 2,3,* ID 1 Department of ENT and Head & Neck Surgery, The Children’s Hospital Zhejiang University School of Medicine, 3333 Bingsheng Road, Hangzhou 310051, China 2 Orthopedic Institute, Soochow University, 708 Remin Road, Suzhou 215006, China; [email protected] (L.L.); [email protected] (R.C.) 3 Shanghai Institute of Traumatology and Orthopaedics, Shanghai Key Laboratory for Prevention and Treatment of Bone and Joint Diseases, Ruijin Hospital, Shanghai Jiao Tong University School of Medicine, 197 Ruijin 2nd Road, Shanghai 200025, China * Correspondence: [email protected] (Y.F.); [email protected] (W.C.); Tel.: +86-571-8667-0860 (Y.F.) Received: 25 January 2018; Accepted: 26 February 2018; Published: 6 March 2018 Abstract: Optimization of nanofiber surface properties can lead to enhanced tissue regeneration outcomes in the context of bone tissue engineering. Herein, we developed a facile strategy to decorate elctrospun nanofibers using extracellular matrix (ECM) in order to improve their performance for bone tissue engineering. Electrospun PLLA nanofibers (PLLA NF) were seeded with MC3T3-E1 cells and allowed to grow for two weeks in order to harvest a layer of ECM on nanofiber surface. After decellularization, we found that ECM was successfully preserved on nanofiber surface while maintaining the nanostructure of electrospun fibers. ECM decorated on PLLA NF is biologically active, as evidenced by its ability to enhance mouse bone marrow stromal cells (mBMSCs) adhesion, support cell proliferation and promote early stage osteogenic differentiation of mBMSCs. -
Self-Assembling Peptides and Their Application in the Treatment of Diseases
International Journal of Molecular Sciences Review Self-Assembling Peptides and Their Application in the Treatment of Diseases Sungeun Lee 1, Trang H.T. Trinh 1, Miryeong Yoo 1, Junwu Shin 1, Hakmin Lee 1, Jaehyeon Kim 1, Euimin Hwang 2, Yong-beom Lim 2 and Chongsuk Ryou 1,* 1 Department of Pharmacy and Institute of Pharmaceutical Science and Technology, Hanyang University, Gyeonggi-do 15588, Korea; [email protected] (S.L.); [email protected] (T.H.T.T.); [email protected] (M.Y.); [email protected] (J.S.); [email protected] (H.L.); [email protected] (J.K.) 2 Department of Materials Science and Engineering, Yonsei University, Seoul 03722, Korea; [email protected] (E.H.); [email protected] (Y.-b.L.) * Correspondence: [email protected]; Tel.: +82-31-400-5811; Fax: +82-31-400-5958 Received: 30 September 2019; Accepted: 20 November 2019; Published: 21 November 2019 Abstract: Self-assembling peptides are biomedical materials with unique structures that are formed in response to various environmental conditions. Governed by their physicochemical characteristics, the peptides can form a variety of structures with greater reactivity than conventional non-biological materials. The structural divergence of self-assembling peptides allows for various functional possibilities; when assembled, they can be used as scaffolds for cell and tissue regeneration, and vehicles for drug delivery, conferring controlled release, stability, and targeting, and avoiding side effects of drugs. These peptides can also be used as drugs themselves. In this review, we describe the basic structure and characteristics of self-assembling peptides and the various factors that affect the formation of peptide-based structures.